The Future Of Quantum Computing What You Need To Know
The Future of Quantum Computing What You Need to Know delves into the exciting and rapidly evolving world of quantum mechanics and its potential impact on various industries. This exploration examines the fundamental principles, current state, and future applications of this revolutionary technology. From understanding qubits and entanglement to exploring the potential for breakthroughs in medicine and materials science, this comprehensive guide provides a concise yet insightful overview.
The landscape of quantum computing is dynamic, and this overview provides a clear picture of the technical aspects, including the hardware, software, and challenges. We’ll also touch on potential ethical implications and the future workforce requirements in this emerging field.
Introduction to Quantum Computing
Quantum computing harnesses the principles of quantum mechanics to perform computations. Unlike classical computers, which rely on bits representing either 0 or 1, quantum computers leverage qubits, capable of existing in a superposition of both states simultaneously. This fundamental difference opens up the possibility of solving complex problems intractable for even the most powerful classical computers.Quantum mechanics describes the behavior of matter and energy at the atomic and subatomic levels.
Key principles include superposition, where a quantum system can exist in multiple states at once, and entanglement, where two or more quantum systems become linked, regardless of the distance separating them. These principles underpin the unique capabilities of quantum computers.
Quantum Mechanics Overview
Quantum mechanics, a branch of physics, governs the behavior of matter at the atomic and subatomic level. Its principles differ significantly from classical mechanics, which describes the macroscopic world. Crucial concepts include the wave-particle duality, where particles exhibit both wave-like and particle-like properties, and the uncertainty principle, highlighting the inherent limitations in knowing certain pairs of physical properties precisely.
The superposition and entanglement of quantum states are pivotal for quantum computing.
Classical vs. Quantum Computing
Classical computers rely on bits, which can represent either 0 or 1. Quantum computers use qubits, which can exist in a superposition of both 0 and 1 simultaneously. This fundamental difference allows quantum computers to explore multiple possibilities concurrently, potentially enabling solutions to problems beyond the reach of classical computers.
Core Concepts: Qubits, Superposition, and Entanglement
Qubits are the fundamental units of quantum information. Unlike classical bits, qubits can exist in a superposition of states, representing both 0 and 1 simultaneously. This allows quantum computers to explore multiple possibilities concurrently, unlike classical computers that examine one possibility at a time. Entanglement is another crucial concept where two or more qubits become linked, meaning their fates are intertwined regardless of the distance separating them.
This correlation enables powerful computational capabilities.
Comparison of Classical and Quantum Bits, The Future of Quantum Computing What You Need to Know
Feature | Classical Bit (bit) | Quantum Bit (qubit) |
---|---|---|
State | 0 or 1 | 0, 1, or a superposition of 0 and 1 |
Information Capacity | 1 bit of information | Potentially much higher due to superposition and entanglement |
Computational Model | Sequential processing | Parallel processing |
Example | A light switch, on or off | A photon’s polarization, exhibiting both horizontal and vertical polarization simultaneously |
Current State of Quantum Computing
Quantum computing, though still in its nascent stages, is rapidly evolving. Researchers are actively developing and improving quantum hardware, algorithms, and software, driving progress toward practical applications. While significant challenges remain, the potential of quantum computing to revolutionize various fields is undeniable.Existing quantum computers, while limited in their current capabilities, offer glimpses into the future of computation. These machines demonstrate the fundamental principles of quantum mechanics, enabling them to tackle problems that are intractable for classical computers.
However, realizing the full potential of quantum computing requires overcoming the technical hurdles that currently constrain their performance.
Examples of Existing Quantum Computers
Several companies and research institutions worldwide are developing and deploying quantum computers. IBM, Google, and Rigetti are prominent examples, offering access to their quantum systems through cloud platforms. These platforms allow researchers and developers to experiment with quantum algorithms and explore the possibilities of this new technology. Specific examples of quantum processors, like the IBM Q System One, demonstrate the physical implementation of quantum bits (qubits).
Limitations and Challenges of Current Quantum Technology
Current quantum computers face significant limitations, primarily stemming from the inherent fragility of quantum states. Quantum bits, or qubits, are highly susceptible to errors caused by environmental noise and decoherence. These errors often limit the size and complexity of problems that can be solved. Furthermore, the current state of quantum hardware often involves a trade-off between the number of qubits and the quality of their control and connectivity.
Controlling and maintaining the delicate quantum states is a major technical challenge.
Progress and Advancements in the Field
Significant progress has been made in the development of quantum computing hardware and software. Improvements in qubit coherence times, gate fidelity, and the scaling of quantum processors are ongoing. Furthermore, the development of new quantum algorithms and techniques continues to expand the capabilities of these machines. These developments are crucial for overcoming the limitations of current technology and paving the way for more powerful and versatile quantum computers.
Types of Quantum Computing Architectures
Different approaches to building quantum computers exist, each with its own strengths and weaknesses. Superconducting qubits, based on the manipulation of electrical currents in superconducting circuits, are a popular choice. Trapped ions, where individual ions are confined and manipulated using lasers, represent another promising architecture. Neutral atoms, where neutral atoms are cooled and trapped in optical lattices, and photonic architectures, which utilize photons to carry quantum information, also form part of the diverse landscape of quantum computing architectures.
Key Characteristics and Performance Metrics of Quantum Computing Platforms
Platform | Architecture | Qubit Count | Coherence Time (µs) | Gate Fidelity (%) |
---|---|---|---|---|
IBM Q System One | Superconducting | ~20 | ~10-100 | ~99 |
Google Sycamore | Superconducting | ~53 | ~10-100 | ~99 |
IonQ | Trapped Ions | ~11 | ~100-1000 | ~99.9 |
Note: The table provides illustrative data. Specific metrics can vary depending on the particular quantum computer and the specific experiment. Values for coherence time and gate fidelity are approximate and may change with time.
Potential Applications of Quantum Computing: The Future Of Quantum Computing What You Need To Know
Quantum computing, with its ability to explore vast solution spaces, promises to revolutionize numerous industries. This potential extends far beyond theoretical concepts, touching upon fields as diverse as medicine and materials science, while presenting new possibilities for financial modeling and beyond. The unique capabilities of quantum algorithms can dramatically accelerate complex calculations, potentially unlocking groundbreaking discoveries and solutions.
Industries Impacted by Quantum Computing
Quantum computing’s impact will be felt across a wide range of industries, from pharmaceuticals to finance. Its capacity to solve intricate problems that are currently intractable for classical computers will lead to substantial advancements. The speed and precision of quantum algorithms will reshape existing processes and create entirely new opportunities.
Impact on Complex Problems
Quantum computing is poised to address problems that are currently beyond the scope of classical computing. These problems often involve simulating complex systems, optimizing intricate processes, and identifying patterns in massive datasets. By leveraging quantum phenomena, quantum algorithms can explore vast solution spaces, potentially leading to breakthroughs in various fields.
Potential Breakthroughs in Specific Fields
Quantum computing presents significant opportunities for breakthroughs in diverse fields. In medicine, it can accelerate drug discovery and personalized medicine. In materials science, it can lead to the design of new materials with enhanced properties. In financial modeling, it can improve risk assessment and portfolio optimization.
Drug Discovery and Development
Quantum computing can dramatically accelerate drug discovery by simulating molecular interactions and predicting drug efficacy. Quantum algorithms can explore the vast chemical space, enabling the identification of potential drug candidates more efficiently than classical methods. This accelerated process could significantly reduce the time and cost associated with bringing new drugs to market.
“Quantum algorithms can simulate molecular interactions with unprecedented accuracy, allowing for the design of novel drugs and therapies.”
Materials Science and Design
Quantum computing can revolutionize materials science by enabling the design of new materials with tailored properties. By simulating the behavior of atoms and molecules, quantum algorithms can predict the properties of materials before they are synthesized, enabling the creation of materials with desired characteristics, such as strength, conductivity, or lightness.
Financial Modeling and Optimization
Quantum computing can enhance financial modeling by optimizing complex financial instruments and portfolios. Quantum algorithms can handle the high dimensionality and computational demands of financial models, potentially improving risk assessment, portfolio optimization, and algorithmic trading strategies.
Table of Potential Applications
Sector | Potential Application | Example |
---|---|---|
Medicine | Drug discovery and design | Simulating molecular interactions to identify potential drug candidates |
Materials Science | Designing new materials | Predicting the properties of new materials before synthesis |
Finance | Portfolio optimization and risk assessment | Optimizing investment portfolios and identifying potential risks |
Chemistry | Catalysis design | Designing catalysts with enhanced efficiency for chemical reactions |
Logistics | Optimizing supply chains | Finding the most efficient routes and schedules for deliveries |
The Future of Quantum Computing Hardware
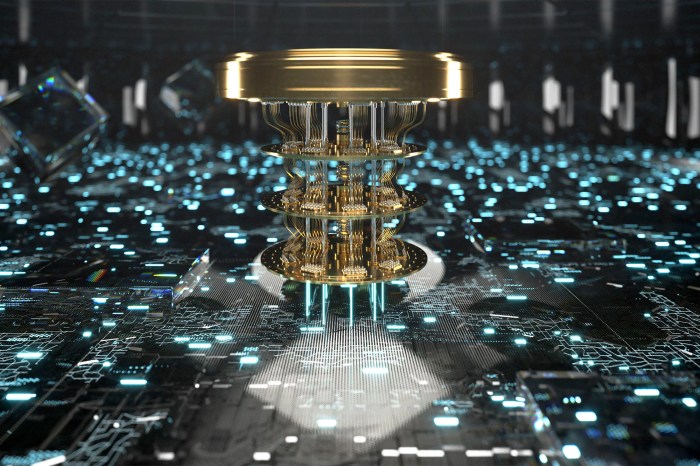
Source: scientificamerican.com
The quest for practical quantum computers is a multifaceted endeavor, pushing the boundaries of current technology. Anticipated advancements in quantum hardware are crucial for realizing the potential of quantum computing, and overcoming current limitations is vital for achieving widespread adoption. This exploration delves into the future landscape of quantum hardware, examining potential solutions, architectures, and the projected timeline for progress.Current quantum computers face significant hurdles, primarily centered around maintaining the delicate quantum states, known as qubits, and connecting them effectively to perform complex computations.
Overcoming these challenges is essential for developing useful quantum algorithms. This includes not only improving qubit stability but also expanding their connectivity to enable larger and more complex computations.
Anticipated Advancements in Quantum Hardware Technology
Quantum hardware advancements are anticipated to focus on several key areas. Improved qubit control and manipulation techniques will be essential for enhancing the fidelity of quantum operations. Enhanced qubit coherence times, crucial for maintaining quantum information, will be a significant target. Increased qubit connectivity, allowing qubits to interact more effectively, will be paramount for enabling more complex computations.
Potential Solutions to Current Limitations
Addressing the critical limitation of decoherence, the loss of quantum information, is a major focus. Quantum error correction techniques are expected to play a pivotal role in mitigating the effects of decoherence. By encoding quantum information across multiple qubits, these techniques can detect and correct errors, effectively protecting the fragile quantum states. Scalability, the ability to build larger and more complex quantum computers, remains a significant challenge.
Innovative qubit architectures and designs will be essential to overcome this barrier.
Future Architectures and Designs for Quantum Computers
Future quantum computer designs are expected to explore various architectures, each with unique strengths and weaknesses. Superconducting qubits, currently a dominant platform, are likely to see improvements in coherence times and scalability. Trapped ions, with their inherent precision, are likely to continue to attract attention in specialized applications. Photonic qubits, relying on light particles, hold the promise of long-distance entanglement and high connectivity.
Neutral atoms, offering excellent control over qubits, might emerge as a promising alternative for larger-scale systems.
The Potential of Quantum Error Correction Techniques
Quantum error correction is a cornerstone for realizing fault-tolerant quantum computation. These techniques provide a crucial mechanism to counteract decoherence, a primary impediment to building large-scale, useful quantum computers. By encoding quantum information redundantly, error correction algorithms can detect and correct errors, ensuring the integrity of computations. This is essential for building large, reliable quantum systems capable of tackling complex problems.
Projected Timeline for Advancements in Quantum Hardware
The timeline for advancements in quantum hardware is dynamic and dependent on various factors, including funding, research breakthroughs, and the development of new materials. While precise timelines are difficult to predict, a steady progression in qubit coherence times, connectivity, and scalability is anticipated within the next decade. Specific milestones, such as achieving fault-tolerant quantum computation, remain uncertain, but continued progress in the field is expected.
Comparison of Quantum Hardware Platforms
Platform | Current State | Predicted Future Developments |
---|---|---|
Superconducting qubits | Dominant platform, achieving reasonable coherence times. | Further improvements in coherence times and scalability through advanced fabrication techniques and circuit designs. |
Trapped ions | High precision, but scalability challenges remain. | Continued development of ion trap technologies and advanced control systems. |
Photonic qubits | Emerging technology, promising long-distance entanglement. | Further exploration of various photonic implementations and improvements in interfacing with other qubit types. |
Neutral atoms | Excellent qubit control, but still under development. | Potential for scalable architectures, but significant challenges in control and connectivity. |
The Future of Quantum Computing Software
Quantum computing’s potential hinges on the development of sophisticated software capable of harnessing the unique capabilities of quantum computers. This involves not only creating algorithms optimized for these machines but also developing the tools and programming languages that empower researchers and developers to effectively utilize them. The journey towards practical quantum computing applications requires significant advancements in software infrastructure.
Development of Quantum Algorithms and Their Importance
Quantum algorithms are central to unlocking the power of quantum computers. They exploit the principles of quantum mechanics to solve problems intractable for classical computers. Algorithms like Shor’s algorithm for factoring large numbers and Grover’s algorithm for searching unsorted databases represent foundational breakthroughs. Continued research and development of novel quantum algorithms are crucial for expanding the scope of problems that can be addressed by quantum computers.
The importance of these algorithms lies in their potential to revolutionize fields like cryptography, materials science, and drug discovery.
Need for Specialized Software Tools and Programming Languages
Quantum computers operate differently from classical computers, demanding specialized software tools and programming languages. Traditional programming languages are ill-equipped to manage the complex quantum states and operations required for quantum computations. Developing languages and tools that directly support quantum algorithms is essential to make quantum computing more accessible and practical.
Potential Impact of Quantum Programming Languages on Software Development
Quantum programming languages are poised to fundamentally reshape software development. They will introduce new paradigms and approaches to problem-solving, fostering innovation in areas like parallel processing and optimization. The emergence of quantum-specific languages could lead to the creation of entirely new software ecosystems and development methodologies.
Examples of Existing and Emerging Quantum Programming Languages
Several quantum programming languages are currently under development. Q# from Microsoft is a prominent example, designed for use with their quantum computing hardware. Other languages, like Cirq from Google and Project Q from IBM, are also gaining traction. These languages offer varying levels of abstraction, making them suitable for different levels of expertise. The future promises a proliferation of quantum programming languages catering to specific needs and applications.
Quantum Software Libraries and Frameworks
Quantum software libraries and frameworks provide essential tools for constructing quantum algorithms and experiments. These libraries often include pre-built modules for common quantum operations, simplifying the development process and fostering collaboration within the community. Open-source frameworks promote the sharing of knowledge and code, accelerating the advancement of quantum computing.
Comparison of Quantum Programming Languages
Programming Language | Features | Strengths | Weaknesses |
---|---|---|---|
Q# | Developed by Microsoft, focuses on .NET ecosystem, strong support for quantum simulators. | Mature ecosystem, good integration with other Microsoft tools. | Limited availability of external libraries, relatively new. |
Cirq | Developed by Google, Python-based, emphasizes experimentation and simulation. | Strong community support, extensive documentation, easy integration with Python libraries. | Limited direct hardware support, more abstract than Q#. |
Project Q | Developed by IBM, Python-based, extensive hardware support. | Strong hardware ties, access to real quantum computers, good community support. | More complex to learn for beginners, evolving ecosystem. |
Challenges and Opportunities for Quantum Computing
Quantum computing, while promising revolutionary advancements, presents a complex landscape of challenges and opportunities. Its potential to solve intricate problems in various fields is undeniable, but its development and deployment must be approached with careful consideration of societal and ethical implications. Navigating these challenges is crucial for realizing the full potential of this transformative technology.
Societal and Ethical Implications
Quantum computing’s potential to revolutionize fields like medicine, materials science, and cryptography necessitates a proactive approach to its ethical and societal impacts. Unforeseen consequences may arise from its use, requiring careful consideration and robust governance frameworks. The development of secure quantum-resistant cryptography is crucial to maintain the security of digital infrastructure in the face of quantum computing’s potential to break existing encryption methods.
Potential societal impacts range from new economic models to shifts in global power dynamics. Furthermore, equitable access to this powerful technology is essential to avoid exacerbating existing inequalities.
Quantum Supremacy and Responsible Development
The concept of quantum supremacy, where a quantum computer outperforms classical computers in specific tasks, raises important concerns. Careful consideration must be given to the potential misuse of such capabilities, including the development of weapons or tools that could be used for malicious purposes. A transparent and rigorous assessment process is necessary to ensure that quantum technology is developed and deployed responsibly.
Furthermore, the development of safety protocols and guidelines is critical to ensure the responsible and beneficial use of quantum computers, especially in the context of potential misuse or unintended consequences.
Collaboration and Education
Addressing the challenges and harnessing the opportunities of quantum computing requires a collaborative effort. Interdisciplinary collaboration among researchers, engineers, policymakers, and ethicists is vital to develop a comprehensive understanding of the technology and its societal impacts. Education and training initiatives are crucial to prepare the workforce for the quantum computing era. This includes promoting STEM education at all levels, fostering interdisciplinary programs, and developing new skill sets needed to effectively utilize quantum computing.
Workforce Needs and Skill Requirements
The quantum computing era will demand a skilled workforce capable of developing, deploying, and utilizing quantum algorithms and hardware. The need for specialized expertise in quantum physics, computer science, and related fields will be paramount. The development of new curricula and training programs is essential to address these needs. These programs should foster interdisciplinary approaches, emphasizing collaboration and adaptability.
Furthermore, ongoing professional development and upskilling will be necessary to keep pace with the rapid advancements in the field.
Ethical Dilemmas and Challenges
Ethical Dilemma | Potential Challenges |
---|---|
Data Privacy and Security | Quantum computers could potentially break existing encryption methods, jeopardizing sensitive data. Developing quantum-resistant cryptography is crucial. |
Access and Equity | High costs of quantum hardware and specialized expertise could lead to unequal access, exacerbating existing societal inequalities. Efforts to democratize access are necessary. |
Misuse of Technology | Quantum computing could be used to develop advanced weapons or tools for malicious purposes. Strict regulations and ethical guidelines are essential. |
Unforeseen Consequences | The potential for unforeseen consequences in diverse fields (e.g., materials science, medicine) necessitates careful monitoring and robust evaluation frameworks. |
Job Displacement | Automation of tasks via quantum computing may lead to job displacement in certain sectors. Reskilling and upskilling initiatives are needed to mitigate these impacts. |
Illustrative Examples of Quantum Computing
Quantum computing, while still in its nascent stages, holds immense promise for tackling complex problems beyond the reach of classical computers. This section provides illustrative examples of how quantum algorithms can be applied to real-world scenarios and how they can outperform classical methods in specific instances. Understanding these examples helps appreciate the potential of quantum computing to revolutionize various fields.
Quantum Algorithm Example: Shor’s Algorithm
Shor’s algorithm is a prime example of a quantum algorithm that can efficiently factor large numbers. Classical algorithms for factoring large numbers are computationally intensive, growing exponentially with the size of the number. This makes them impractical for very large numbers. Shor’s algorithm, however, leverages quantum phenomena to perform this task significantly faster.
Factoring a 1024-bit number, a task currently considered infeasible for classical computers, might take a quantum computer only a few hours.
This drastically impacts fields like cryptography, where the security of widely used public-key encryption relies on the difficulty of factoring large numbers. A quantum computer capable of implementing Shor’s algorithm could potentially break these encryption schemes.
Real-World Use Cases
Quantum computing’s potential spans various sectors. In materials science, it could accelerate the discovery of new materials with specific properties, leading to breakthroughs in energy storage, medicine, and electronics. In finance, it could optimize investment strategies, leading to potentially higher returns and more effective risk management.
Quantum Computing and Optimization Problems
Quantum computers excel at optimization problems, where the goal is to find the best solution from a vast search space. For instance, designing the most efficient layout for a microchip or finding the optimal routes for a fleet of delivery vehicles are optimization challenges where quantum algorithms can offer advantages. Quantum annealing algorithms, specifically designed for optimization, are already being explored for practical applications.
Illustrative Examples of Quantum Algorithms
Several quantum algorithms are being developed to solve specific problems. One example is the Grover’s algorithm, which can speed up searching an unsorted database. This could be crucial in large databases where finding a specific record would take a long time with classical algorithms.
- Grover’s algorithm: Offers a quadratic speedup over classical search algorithms. This is significant in scenarios with large datasets, where finding a particular element becomes computationally intensive with traditional methods.
- Quantum Approximate Optimization Algorithm (QAOA): A versatile algorithm for tackling complex optimization problems in diverse fields, such as logistics, supply chain management, and drug discovery.
Performance Comparison: Classical vs. Quantum Algorithms
The following table illustrates a hypothetical scenario comparing the performance of classical and quantum algorithms for a specific optimization problem.
Algorithm | Time Complexity (Hypothetical Example) | Problem Size |
---|---|---|
Classical (Brute-Force) | O(2n) | n = 50 |
Quantum (QAOA) | O(n2) | n = 50 |
The table demonstrates a potential exponential speedup for quantum algorithms in this specific scenario. However, it’s crucial to remember that these are simplified examples and the practical performance can vary greatly based on the specifics of the problem and the available quantum hardware.
Final Thoughts
In conclusion, the future of quantum computing promises significant advancements across numerous sectors. While challenges remain, the potential benefits are vast, and the ongoing research and development will shape the future in profound ways. Understanding the basics, current state, and projected developments is crucial for anyone seeking to navigate this fascinating and rapidly changing field.
Post Comment