Future Of Quantum Computing Technology Applications
Future of quantum computing technology applications promises a revolution across various sectors. This exploration delves into the exciting potential of quantum computing, examining its fundamental principles, current state, and emerging applications. From healthcare breakthroughs to advanced materials, the technology’s potential to transform our world is immense.
The Artikel explores quantum computing’s core concepts like superposition and entanglement, contrasting them with classical computing. It analyzes different architectures, including superconducting and trapped ions, providing a comprehensive overview of the current landscape. Furthermore, it dissects the challenges, limitations, and algorithms underpinning this revolutionary technology.
Introduction to Quantum Computing
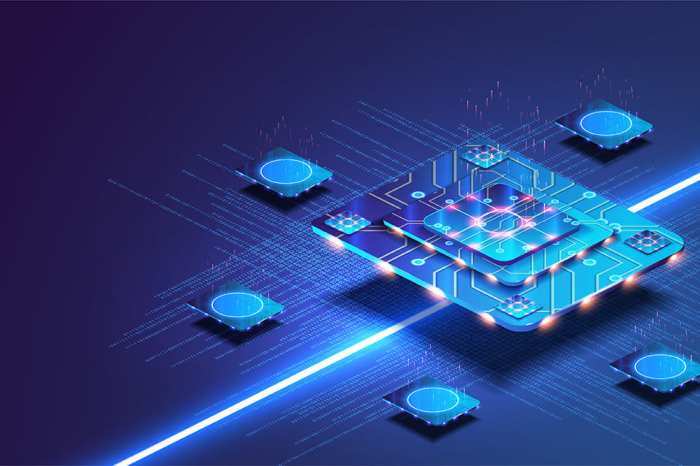
Source: bernardmarr.com
Quantum computing represents a paradigm shift in computing, leveraging the principles of quantum mechanics to perform calculations in ways fundamentally different from classical computers. This approach promises to solve problems currently intractable for even the most powerful classical supercomputers, opening doors to advancements in fields ranging from materials science to drug discovery. The core concepts of superposition and entanglement are central to this revolution.Quantum mechanics allows particles to exist in multiple states simultaneously, a phenomenon known as superposition.
This contrasts sharply with classical bits, which are either 0 or 1. A quantum bit, or qubit, can represent both 0 and 1 simultaneously, exponentially increasing the computational power available. Entanglement further enhances this power by linking multiple qubits, allowing them to be correlated in ways that transcend classical correlations. This interconnectedness allows for complex computations that are impossible to perform with classical methods.
Quantum Computing Principles
Quantum computing leverages the principles of superposition and entanglement to perform calculations. Superposition allows qubits to exist in multiple states simultaneously, significantly increasing the computational space. Entanglement links multiple qubits in a correlated way, enabling complex computations.
Classical vs. Quantum Computing
Classical computers rely on bits, which are either 0 or 1. Quantum computers, in contrast, utilize qubits, which can represent both 0 and 1 simultaneously through superposition. This fundamental difference allows quantum computers to explore multiple possibilities concurrently, leading to exponential speedups for certain types of problems. The mathematical underpinnings of quantum mechanics, including the Schrödinger equation, are crucial to designing and executing quantum algorithms.
Current State of Quantum Computing Technology
Quantum computing technology is currently in its nascent stages. While significant progress has been made in developing quantum processors, these devices are still prone to errors. Quantum error correction techniques are being developed to mitigate these issues, aiming to build more stable and reliable quantum computers. The development of fault-tolerant quantum computers is a key objective for the field.
Current research focuses on optimizing qubit control, reducing decoherence, and developing efficient quantum algorithms. The development of more sophisticated quantum algorithms tailored to specific problems is crucial for realizing the full potential of quantum computing.
Quantum Computing Architectures
Different approaches exist for building quantum computers, each with its own strengths and limitations. The choice of architecture depends on the specific problem to be solved. A comparison of key quantum computing architectures is presented below.
Architecture | Description | Strengths | Limitations |
---|---|---|---|
Superconducting | Uses superconducting circuits to create and manipulate qubits. | Scalability, relatively mature technology. | Susceptibility to noise, limited coherence times. |
Trapped Ions | Uses electromagnetic fields to trap and manipulate atomic ions. | High fidelity, long coherence times. | Scalability challenges, complex fabrication. |
Photonic | Uses photons to encode and process quantum information. | Potential for long-distance communication, low interaction strength. | High experimental complexity, challenges in controlling entanglement. |
Emerging Applications in Diverse Sectors
Quantum computing’s potential extends far beyond theoretical concepts. Its unique capabilities are poised to revolutionize various sectors, offering solutions to complex problems previously intractable for classical computers. This section explores the exciting possibilities in healthcare, materials science, and finance, highlighting the transformative impact this technology promises.Quantum algorithms can tackle tasks that are practically impossible for current computers, offering a powerful new approach to problem-solving.
These capabilities are already being explored and tested, leading to promising advancements across different industries.
Quantum Computing in Healthcare
Quantum computing holds significant promise for accelerating drug discovery and personalizing medicine. The ability to simulate molecular interactions at an unprecedented level allows for the design of novel drugs and therapies with enhanced efficacy and reduced side effects. Quantum algorithms can quickly analyze vast datasets of patient information, enabling the development of more accurate diagnostic tools and personalized treatment plans.
- Drug Discovery: Quantum computers can simulate the behavior of molecules with unprecedented accuracy, enabling the design of new drugs and materials with desired properties. This process is often computationally intensive and time-consuming for classical computers. Quantum algorithms can drastically reduce the time required for drug development.
- Personalized Medicine: Quantum computing can analyze patient genetic data to identify specific genetic markers and predict individual responses to different therapies. This approach allows for tailored treatment plans, leading to improved patient outcomes and reduced trial-and-error processes.
Quantum Computing in Materials Science and Engineering
Quantum computing can be a powerful tool in materials science and engineering, accelerating the discovery of novel materials with specific properties. The ability to simulate complex atomic interactions allows for the design of materials with tailored functionalities for diverse applications. This capability has implications for advancements in energy storage, aerospace, and electronics.
- Designing Novel Materials: Quantum simulations can predict the properties of materials at the atomic level, allowing scientists to design new materials with enhanced properties. This accelerates the development of materials for applications like stronger and lighter alloys or more efficient solar cells.
- Optimization of Existing Materials: Quantum computing can optimize the structure and composition of existing materials to improve their performance in specific applications. This could lead to enhanced efficiency in various sectors.
Quantum Computing in Financial Modeling and Risk Assessment
Quantum algorithms offer potential improvements in financial modeling and risk assessment, particularly in complex scenarios involving large datasets and intricate interactions. The speed and efficiency of quantum computing could lead to more accurate risk assessments, portfolio optimization, and fraud detection.
- Financial Modeling: Quantum algorithms can solve complex optimization problems in finance, such as portfolio optimization and risk management. This can lead to more efficient allocation of resources and better risk mitigation strategies.
- Fraud Detection: Quantum algorithms can identify patterns and anomalies in large datasets, enhancing the effectiveness of fraud detection systems in financial institutions. The speed and accuracy of quantum algorithms could lead to quicker identification of suspicious transactions.
Advantages and Disadvantages of Quantum Computing in Various Sectors
Sector | Advantages | Disadvantages |
---|---|---|
Healthcare | Accelerated drug discovery, personalized medicine, improved diagnostics | High cost of quantum computers, limited availability of trained personnel |
Materials Science | Design of novel materials with tailored properties, optimization of existing materials | Complex simulations may require specialized quantum algorithms, interpretation of results |
Finance | Improved risk assessment, more accurate portfolio optimization, faster fraud detection | Security concerns related to quantum algorithms, potential impact on existing financial models |
Challenges and Limitations of Quantum Computing
Quantum computing, while promising revolutionary advancements, faces significant hurdles in its development and practical implementation. These limitations stem from the very nature of quantum mechanics, which requires extremely controlled environments to maintain the delicate quantum states. Overcoming these challenges is crucial for realizing the full potential of quantum computing.
Technological Hurdles in Building and Maintaining Stable Quantum Computers
Quantum computers require exceptionally controlled environments to maintain the delicate quantum states of qubits. These states are highly susceptible to disturbances from the surrounding environment, leading to errors. Maintaining coherence, the ability of a quantum system to retain its quantum properties, is a significant hurdle. Minimizing these environmental effects is essential for accurate computations. Specialized materials and designs are needed to create stable and isolated qubit environments.
For instance, superconducting qubits are often cooled to near absolute zero temperatures to minimize thermal fluctuations. These extreme conditions pose significant engineering challenges in terms of cooling and isolation.
Qubit Coherence and Error Correction
Qubit coherence, a crucial aspect of quantum computation, is the ability of a qubit to retain its quantum properties over time. Decoherence, the loss of quantum properties, is a primary limitation, impacting the accuracy and duration of computations. Quantum algorithms are highly sensitive to errors, demanding robust error correction strategies. Current error correction techniques are still under development and require substantial advancements to handle the complex errors inherent in large-scale quantum systems.
The development of more sophisticated error correction codes is essential for reliable computations. For example, fault-tolerant quantum computation aims to mitigate errors, ensuring that computations are accurate even with imperfections.
Scaling Up Quantum Computing Systems
Scaling up quantum computing systems to handle complex problems presents a major challenge. The number of qubits needed to solve practical problems is often significantly higher than what current systems can offer. Connecting and controlling numerous qubits while maintaining coherence is a complex engineering problem. Current systems are limited in terms of the number of qubits and the complexity of quantum circuits they can execute.
As the number of qubits increases, the complexity of maintaining coherence and controlling interactions between them also increases exponentially.
Current Limitations of Quantum Computing Hardware and Software
Category | Limitation | Impact |
---|---|---|
Hardware | Qubit coherence time | Limited computation duration and accuracy |
Hardware | Qubit connectivity | Difficulty in creating complex quantum circuits |
Hardware | Scalability | Inability to handle problems requiring a large number of qubits |
Software | Algorithm development | Limited range of solvable problems |
Software | Error mitigation | Computational results can be unreliable |
Software | Quantum software ecosystem | Limited tools and expertise |
Current quantum computers are often limited in terms of qubit coherence times, which determine the length of computation. The connectivity of qubits also impacts the complexity of quantum circuits. Furthermore, the scalability of quantum computing hardware is still in its infancy, limiting the size of problems that can be tackled. The development of efficient quantum algorithms remains a significant challenge, limiting the types of problems quantum computers can solve.
In addition, robust error correction strategies are needed to guarantee accurate results in the face of errors. Lastly, a robust quantum software ecosystem is crucial to enable the widespread adoption of quantum computing.
Quantum Algorithms and Their Applications
Quantum algorithms leverage the unique properties of quantum mechanics to solve problems intractable for classical computers. These algorithms offer the potential to revolutionize various fields by providing solutions to complex challenges currently beyond the capabilities of classical computing. Their impact will be significant, ranging from breaking current encryption methods to optimizing complex logistical problems.
Shor’s Algorithm and Cryptography
Shor’s algorithm, a landmark development in quantum computing, poses a significant threat to current cryptographic systems. Its ability to efficiently factor large numbers has profound implications for the security of widely used encryption methods. This algorithm exploits the principles of quantum superposition and entanglement to dramatically speed up the process of factoring compared to classical algorithms.
Shor’s algorithm provides a polynomial-time solution to the integer factorization problem, a problem that is considered intractable for classical computers using known algorithms.
The potential impact on cryptography is immense. Systems relying on the difficulty of factoring large numbers, like RSA, could become vulnerable. This necessitates the development of new cryptographic methods resistant to quantum attacks. Research is actively underway to explore post-quantum cryptography, which seeks to develop algorithms secure against attacks from quantum computers.
Grover’s Algorithm and Optimization Problems
Grover’s algorithm offers a significant advantage in solving optimization problems. It’s a quantum search algorithm that can quadratically speed up the search for solutions compared to classical search methods. This is particularly useful in finding solutions in large, unstructured datasets, where the number of possible solutions is vast.
Grover’s algorithm achieves a quadratic speedup over classical search algorithms, making it particularly valuable in large-scale optimization problems.
Applications span diverse fields, including logistics, financial modeling, and drug discovery. For instance, in logistics, it could optimize delivery routes or resource allocation. In financial modeling, it could accelerate portfolio optimization. In drug discovery, it could expedite the identification of promising drug candidates.
Quantum Algorithms and Their Applications – Table
Algorithm | Problem Domain | Key Benefit | Example Application |
---|---|---|---|
Shor’s Algorithm | Integer factorization, discrete logarithm | Exponential speedup over classical algorithms | Breaking RSA encryption |
Grover’s Algorithm | Unstructured search | Quadratic speedup over classical algorithms | Optimizing delivery routes, drug discovery |
Quantum Approximate Optimization Algorithm (QAOA) | Optimization problems | Provides a heuristic approach to complex optimization problems | Designing materials with desired properties, financial portfolio optimization |
Variational Quantum Eigensolver (VQE) | Finding ground states of molecules | Accurate calculation of ground states of molecules | Developing new materials, designing catalysts |
Future Directions and Research Trends
The quantum computing landscape is rapidly evolving, promising transformative advancements across diverse sectors. Anticipated progress in hardware, software, and integration with existing technologies will be crucial for realizing the full potential of this revolutionary field. The next decade is poised to witness significant breakthroughs, leading to more powerful and accessible quantum computers.The quest for practical quantum computing is driving a flurry of research initiatives.
Researchers are actively exploring novel approaches to improve qubit stability, enhance quantum gates’ efficiency, and scale up quantum systems. This, in turn, will lead to the development of more robust and versatile quantum algorithms capable of tackling complex problems in various fields.
Anticipated Advancements in Quantum Computing Technology
Quantum computers are expected to become more powerful, with increased qubit counts and reduced error rates. This advancement will be driven by improvements in qubit fabrication and control, enabling the execution of more complex algorithms. For example, current efforts to create more stable and longer-lived qubits will lead to quantum computers capable of handling larger problem sizes and more sophisticated computations.
Furthermore, improvements in quantum error correction techniques will minimize errors during computation, leading to more reliable and accurate results.
Evolving Research Directions in Quantum Computing Hardware
Research in quantum computing hardware focuses on several key areas. First, development of novel qubit types, including trapped ions, superconducting circuits, and photonic qubits, will continue. Each approach has its strengths and weaknesses, and researchers are actively exploring the trade-offs. Second, enhanced qubit control methods are crucial to maintain and manipulate qubits efficiently. Advancements in control techniques are crucial to precisely manipulating and measuring qubits, which is essential for reliable computation.
Third, the design of quantum architectures and scalable designs will be key to building quantum computers with a large number of qubits. These designs will focus on minimizing qubit-qubit interactions and optimizing resource utilization.
Evolving Research Directions in Quantum Computing Software, Future of quantum computing technology applications
Quantum software development is rapidly progressing. Researchers are focusing on developing new quantum algorithms for specific applications, creating specialized tools for quantum programming, and enhancing quantum software development frameworks. For instance, designing algorithms that are tailored to exploit the unique characteristics of different quantum hardware architectures is essential. Further development of quantum programming languages and tools will simplify the development process, attracting a wider range of users.
Optimization algorithms tailored for quantum computing will also become more prevalent.
Integration of Quantum Computing with Other Technologies
Quantum computing is poised to revolutionize several industries by integrating with other technologies. For example, integration with machine learning will lead to more sophisticated models for data analysis and prediction. Combining quantum computing with artificial intelligence (AI) will enable the development of hybrid algorithms that leverage the strengths of both technologies. Furthermore, the integration of quantum computing with materials science will allow for the design and discovery of novel materials with enhanced properties.
Future Research Priorities in Quantum Computing
Research Area | Priority Level | Description |
---|---|---|
Qubit Stability and Control | High | Improving qubit coherence times and developing robust control methods to minimize errors and increase computation time. |
Quantum Error Correction | High | Developing and implementing efficient error correction protocols to enhance the reliability of quantum computations. |
Quantum Algorithms and Applications | High | Developing new algorithms tailored for specific problems in various fields, focusing on applications like drug discovery, materials science, and financial modeling. |
Quantum Software Development Tools | High | Developing user-friendly programming languages, simulators, and tools to simplify quantum algorithm design and implementation. |
Quantum Architectures and Scalability | High | Designing scalable quantum architectures that can accommodate a large number of qubits and integrate different qubit types. |
Societal Impacts and Ethical Considerations
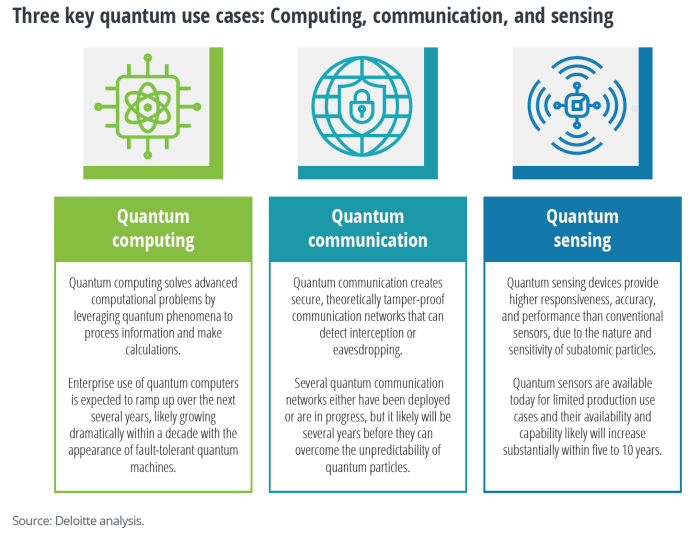
Source: amazonaws.com
Quantum computing promises transformative potential across various sectors, yet its implementation necessitates careful consideration of the societal and ethical implications. This technology’s unprecedented computational power could revolutionize fields from medicine to materials science, but it also presents novel challenges regarding privacy, security, and the equitable distribution of its benefits.The societal impact of quantum computing hinges on responsible development and deployment.
Understanding both the positive and negative consequences is crucial for shaping its trajectory and ensuring it serves humanity’s best interests. This involves navigating ethical dilemmas, ensuring equitable access, and mitigating potential risks.
Potential Societal Impacts
The development and deployment of quantum computing hold the promise of significant positive impacts on society, from accelerating scientific breakthroughs to addressing critical global challenges. However, it is essential to acknowledge potential negative consequences and implement safeguards to mitigate risks.
- Positive Impacts: Quantum computing has the potential to revolutionize various fields, including drug discovery, materials science, and financial modeling. Faster and more efficient drug discovery can lead to new treatments and cures for diseases. Advancements in materials science could lead to the development of stronger, lighter, and more efficient materials for diverse applications. Quantum algorithms can optimize financial models, potentially improving investment strategies and reducing risks.
- Negative Impacts: The rapid advancement of quantum computing raises concerns about potential misuse. For example, quantum algorithms could potentially break current encryption methods, jeopardizing sensitive information and national security. Furthermore, the economic impact of quantum computing could lead to job displacement in certain sectors, necessitating proactive measures for workforce retraining and adaptation.
Ethical Implications in Privacy and Security
The ability of quantum computers to break current encryption methods poses a significant threat to digital security and privacy. Developing post-quantum cryptography is crucial to safeguarding sensitive data.
- Quantum Computing and Encryption: Quantum computers have the potential to break widely used encryption algorithms, which could compromise sensitive data like financial transactions, medical records, and government communications. This necessitates the development and implementation of post-quantum cryptography to protect against these vulnerabilities.
- Data Privacy: The increased processing power of quantum computers could facilitate the analysis of vast datasets, potentially leading to more sophisticated data mining and profiling. Careful consideration of data privacy and the responsible use of quantum-enabled data analysis tools is essential to prevent misuse.
Examples of Solving Global Challenges
Quantum computing offers the potential to address pressing global challenges through various applications. For example, optimized energy grids, more efficient resource management, and improved climate modeling are possible outcomes.
- Climate Change Mitigation: Quantum simulations can model complex climate systems with unprecedented accuracy, potentially aiding in the development of more effective strategies for mitigating climate change.
- Drug Discovery and Development: Quantum computing can accelerate the discovery and development of new drugs, leading to more effective treatments and cures for diseases.
- Financial Modeling: Quantum algorithms can optimize financial models, potentially reducing risk and improving investment strategies.
Potential Benefits and Risks of Quantum Computing on Society
The following table summarizes the potential benefits and risks associated with the deployment of quantum computing.
Aspect | Potential Benefits | Potential Risks |
---|---|---|
Security | Development of new, quantum-resistant encryption methods. | Vulnerability of current encryption methods to quantum attacks. |
Economy | Creation of new industries and jobs related to quantum computing. | Job displacement in sectors impacted by automation. |
Healthcare | Faster drug discovery and development. | Potential for misuse of sensitive health data. |
Environment | Optimized energy grids and resource management. | Potential for increased energy consumption by quantum computers. |
Quantum Computing Infrastructure and Development: Future Of Quantum Computing Technology Applications
The burgeoning field of quantum computing necessitates a robust infrastructure to support its development and application. This infrastructure encompasses not only the physical hardware, but also the software tools, algorithms, and the supporting research and development ecosystem. Progress in this area is critical to realizing the potential of quantum computing across diverse sectors.The global quantum computing landscape is still nascent, yet significant strides are being made.
While the technology is still largely experimental, efforts are focused on building more stable and powerful quantum computers. This includes improving qubit coherence times, error rates, and the scalability of quantum systems. The journey toward practical quantum computing is marked by continuous innovation and collaboration across academia and industry.
Current Quantum Computing Infrastructure
The current global quantum computing infrastructure is diverse, ranging from small-scale systems accessible through cloud platforms to larger, more specialized machines developed by leading research institutions and companies. Access to these systems is often limited, primarily due to their experimental nature and high cost. However, increasing accessibility is a key factor in driving the field forward. Early adoption and experimentation are vital for developing practical applications and for identifying the unique strengths and limitations of these technologies.
Government Funding and Private Investment
Government funding plays a crucial role in supporting quantum computing research and development. Countries recognizing the transformative potential of this technology are investing heavily in research initiatives, aiming to develop cutting-edge quantum hardware and software. Private investment is also vital, as it drives the development of commercial applications and facilitates the creation of new companies focused on quantum computing technologies.
This collaborative funding model fosters innovation and ensures the field maintains momentum.
Leading Companies and Research Institutions
Numerous companies and research institutions are actively involved in the development of quantum computing technology. Some prominent examples include IBM, Google, and Rigetti, leading the charge in hardware development. Companies like Honeywell and IonQ are focused on building and commercializing quantum computing systems, with different approaches to qubit technology. Academic institutions like MIT, Caltech, and the University of Oxford are contributing to fundamental research, developing new algorithms and exploring theoretical aspects.
This collective effort is essential for the future of quantum computing.
- IBM: Known for its cloud-based quantum computing platforms, offering access to various quantum processors to researchers and developers.
- Google: Has been at the forefront of achieving quantum supremacy, demonstrating the potential of quantum computers to solve specific problems beyond the reach of classical computers.
- Rigetti: Focuses on superconducting qubit technology, aiming to build high-performance quantum computers.
- Honeywell: Emphasizes trapped-ion technology, a different approach to qubit construction.
- IonQ: A company specializing in trapped-ion quantum computing systems.
- MIT, Caltech, Oxford: Renowned universities actively contributing to quantum computing research and development through fundamental research, algorithm development, and theoretical exploration.
Funding and Investment Trends
The quantum computing industry is experiencing rapid growth in funding and investment. This trend reflects the significant potential of the technology and the growing recognition of its impact on various sectors. Early-stage funding is particularly important for nurturing innovation and driving progress.
Year | Funding Type | Estimated Amount (USD) | Source |
---|---|---|---|
2022 | Venture Capital | $1 Billion | (Source: Estimated based on publicly available data and market analysis reports) |
2023 | Government Grants | $500 Million | (Source: Estimated based on publicly available government funding announcements and research reports) |
2024 | Private Equity | $750 Million | (Source: Estimated based on anticipated investment trends and market projections) |
Note: Funding figures are approximate and may vary based on different data sources and methodologies.
Quantum Computing in Artificial Intelligence
Quantum computing presents a revolutionary opportunity to enhance artificial intelligence (AI) algorithms and capabilities, promising breakthroughs in areas like machine learning, natural language processing, and computer vision. The inherent parallelism and superposition properties of quantum systems allow for the exploration of exponentially larger solution spaces than classical computers, potentially leading to more efficient and accurate AI models.The potential of quantum computing to accelerate AI development is significant.
By leveraging quantum algorithms, researchers aim to build AI systems that can learn from data more quickly, identify complex patterns more efficiently, and make more accurate predictions. This enhanced capability is expected to unlock significant advancements in numerous sectors, from healthcare and finance to scientific discovery and entertainment.
Quantum Enhancement of AI Algorithms
Quantum computing offers a paradigm shift in AI algorithm design. Quantum algorithms, like quantum machine learning (QML) algorithms, exploit quantum phenomena to perform complex computations faster than classical algorithms. This speedup can be particularly beneficial in tasks requiring large-scale data analysis and complex pattern recognition, both key components in many AI applications. The potential for quantum computing to solve complex optimization problems in machine learning is particularly exciting.
Quantum Machine Learning
Quantum machine learning (QML) algorithms are designed to leverage the unique capabilities of quantum computers for AI tasks. These algorithms aim to solve problems such as feature extraction, model training, and classification more efficiently than their classical counterparts. This leads to faster learning and potentially more accurate predictions. QML algorithms could find application in areas like drug discovery, materials science, and financial modeling, where large datasets and complex relationships need to be analyzed.
Impact on Natural Language Processing and Computer Vision
Quantum computing’s impact extends to natural language processing (NLP) and computer vision (CV). In NLP, quantum algorithms could enable faster and more accurate language understanding and translation, facilitating more nuanced interactions with machines. In computer vision, quantum algorithms could accelerate image recognition and analysis, leading to more sophisticated image processing and potentially enabling new applications like autonomous driving.
Integration of Quantum Computing with AI Techniques
AI Technique | Quantum Computing Integration | Potential Benefits |
---|---|---|
Machine Learning | Quantum machine learning algorithms (QML) | Faster training, improved accuracy, handling larger datasets. |
Deep Learning | Quantum neural networks, quantum optimization for deep learning architectures | Potential for faster convergence, improved model accuracy, especially in high-dimensional spaces. |
Natural Language Processing | Quantum algorithms for text processing, language understanding | More accurate language models, faster language translation, and enhanced understanding of complex linguistic structures. |
Computer Vision | Quantum algorithms for image analysis, pattern recognition | Faster image processing, more accurate object detection, potentially leading to improved image recognition and analysis tasks. |
Reinforcement Learning | Quantum algorithms for optimization in reinforcement learning | Potentially faster learning in complex environments, more effective exploration of state spaces. |
Last Recap
In conclusion, the future of quantum computing technology applications is brimming with potential, offering transformative solutions across diverse fields. While significant challenges remain, the relentless pursuit of innovation and collaboration promises to unlock unprecedented advancements. The integration of quantum computing with other technologies, coupled with ongoing research, will likely shape a future where complex problems are tackled with unparalleled efficiency and accuracy.
Post Comment