Solid-State Batteries Smartphone Mobile Power Future
Solid-state batteries smartphone mobile power future holds immense potential for revolutionizing portable electronics. These batteries, distinguished by solid electrolytes, promise superior performance compared to lithium-ion counterparts. They could dramatically improve smartphone battery life, charging speeds, and overall power delivery. This exploration delves into the scientific underpinnings, practical applications, and future prospects of solid-state battery technology.
From enhanced energy and power densities to improved safety profiles, solid-state batteries are poised to reshape the mobile power landscape. This discussion explores the technical hurdles, cost implications, and societal impact of this transformative technology. It examines how these batteries can enhance performance in diverse mobile applications, including smartphones, and other portable devices.
Introduction to Solid-State Batteries
Solid-state batteries represent a significant advancement in energy storage technology, promising substantial improvements over existing lithium-ion batteries. Their core innovation lies in replacing the flammable liquid electrolyte with a solid material, leading to enhanced safety, potentially higher energy density, and improved performance characteristics. This shift holds the potential to revolutionize various sectors, from electric vehicles to portable electronics.Solid-state batteries leverage a solid electrolyte to facilitate ion transport between the positive and negative electrodes.
This solid electrolyte, often composed of ceramic or polymer materials, offers superior safety and stability compared to liquid electrolytes, which are susceptible to leakage and thermal runaway. Furthermore, the solid electrolyte structure can potentially enable higher operating temperatures, leading to improved energy density and faster charging times.
Key Characteristics of Solid-State Batteries
Solid-state batteries are distinguished by their solid electrolyte, which plays a crucial role in their overall performance. This unique feature allows for greater safety, improved thermal stability, and enhanced power delivery compared to traditional lithium-ion batteries. The solid nature of the electrolyte mitigates the risk of short circuits and thermal runaway, making them potentially safer for use in various applications.
Fundamental Scientific Principles
The fundamental principle behind solid-state batteries revolves around the controlled movement of lithium ions through the solid electrolyte. This process is crucial for efficient energy storage and delivery. The solid electrolyte acts as a medium to transport lithium ions from the negative electrode to the positive electrode, enabling the flow of electrical current. The design and properties of the solid electrolyte directly influence the battery’s overall performance, including energy density, power density, and cycle life.
The ionic conductivity of the solid electrolyte is a critical parameter, as it dictates how readily lithium ions can move within the material. High ionic conductivity is essential for fast charging and high power delivery.
Types of Solid Electrolytes
Different types of solid electrolytes are being investigated for use in solid-state batteries, each with its unique properties. Ceramic electrolytes, such as lithium lanthanum titanate (LLTO), are known for their high ionic conductivity at higher temperatures. Polymer electrolytes, on the other hand, exhibit flexibility and potentially lower manufacturing costs.
- Ceramic Electrolytes: Ceramic electrolytes, often oxides or nitrides, offer high ionic conductivity at higher temperatures, making them potentially suitable for applications like electric vehicles where higher operating temperatures are more common. Examples include lithium lanthanum titanate (LLTO) and lithium sulfide (Li 2S). The high ionic conductivity at higher temperatures can translate into better performance in high-temperature environments.
- Polymer Electrolytes: Polymer electrolytes, composed of a polymer matrix with a dissolved lithium salt, exhibit flexibility, potentially reducing manufacturing costs. They often require additives to enhance ionic conductivity. However, their ionic conductivity is generally lower compared to ceramic electrolytes.
Performance Comparison
A comparative analysis of performance metrics between solid-state batteries and lithium-ion batteries reveals potential advantages for the former.
Metric | Solid-State Battery | Lithium-Ion Battery |
---|---|---|
Energy Density (Wh/kg) | Potentially higher | Moderate |
Power Density (W/kg) | Potentially higher | Moderate |
Cycle Life (cycles) | Potentially higher | Moderate |
Note: The potential values for solid-state batteries are based on ongoing research and development. Actual performance may vary depending on specific material compositions and manufacturing processes.
Solid-State Batteries in Smartphones
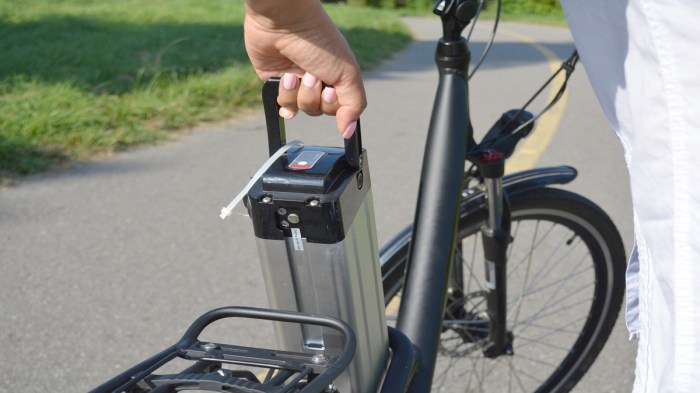
Source: market-prospects.com
Solid-state batteries, a promising advancement in energy storage technology, hold the potential to revolutionize various electronic devices, including smartphones. Their unique characteristics, such as enhanced safety and potentially higher energy density, make them an exciting prospect for the future of portable electronics. This section will delve into the specifics of solid-state batteries in smartphones, examining their advantages, limitations, and integration challenges.
Potential Benefits of Solid-State Batteries
Solid-state batteries offer several compelling advantages over their lithium-ion counterparts, particularly for smartphones. Improved performance metrics, such as extended battery life and faster charging times, are expected outcomes. The reduced form factor is another key benefit, allowing for thinner and lighter devices without sacrificing battery capacity. These factors are crucial for enhancing the user experience and market appeal of smartphones.
Comparison with Lithium-Ion Batteries
Lithium-ion batteries, currently dominant in the smartphone market, suffer from limitations in terms of safety, charging speed, and overall performance. They are susceptible to overheating and fire hazards, which are critical safety concerns. Furthermore, their charging times can be relatively slow, and their energy density is not as high as potentially achievable with solid-state technology. Solid-state batteries, with their inherent safety and high energy density, are poised to address these limitations.
Challenges of Integration
Integrating solid-state batteries into existing smartphone designs presents a number of challenges. The differing physical properties of solid-state electrolytes compared to liquid electrolytes necessitate significant design modifications. Existing manufacturing processes for lithium-ion battery production might need substantial adaptation for solid-state systems. Compatibility issues with existing smartphone charging infrastructure and electronic components also need to be addressed.
Technical Requirements for Manufacturing
Several technical requirements need to be met to produce solid-state batteries suitable for smartphones. The development of robust, high-performance solid electrolytes is paramount. These electrolytes must exhibit excellent ionic conductivity while maintaining high mechanical stability and chemical inertness. The manufacturing process must also be scalable and cost-effective to ensure affordability. Furthermore, the cell design must be optimized for the specific requirements of smartphone applications.
Potential Improvements in Performance Metrics
Feature | Lithium-Ion Battery (Estimated) | Solid-State Battery (Potential) |
---|---|---|
Battery Life (hours) | 10-12 hours | 14-18 hours |
Charging Time (hours) | 1-2 hours | 30 minutes-1 hour |
Form Factor (mm) | 5-7 mm | 4-6 mm |
Safety | Moderate risk of thermal runaway and fire hazards | Minimized or virtually eliminated risk |
These potential improvements are based on projected advancements in solid-state battery technology. Factors such as manufacturing costs, material availability, and regulatory approvals will ultimately influence the actual adoption timeframe. Real-world examples of battery technology improvements, such as the transition from nickel-cadmium to lithium-ion batteries in consumer electronics, illustrate the transformative potential of new battery chemistries.
Mobile Power Applications
Solid-state batteries promise a revolution in mobile power, offering significant advantages over conventional lithium-ion batteries. Their enhanced safety profile, higher energy density, and potentially faster charging times make them attractive for a wide range of mobile applications. This section explores the specific applications and how solid-state batteries can improve performance and efficiency.
Potential Benefits for Portable Electronics
Solid-state batteries, with their higher energy density, can enable smaller, lighter, and more powerful portable electronics. This translates to longer battery life in smartphones, tablets, laptops, and other devices, without compromising the device’s overall size. The enhanced safety features of solid-state batteries could also lead to more robust and durable portable electronics, reducing the risk of fire or other safety hazards associated with lithium-ion batteries.
Furthermore, faster charging times are anticipated, reducing the downtime required for recharging.
Applications in Electric Vehicles
Solid-state batteries’ high energy density and improved safety characteristics are well-suited for electric vehicles (EVs). This allows for greater driving range and faster charging times compared to lithium-ion batteries. Their ability to withstand higher operating temperatures could also enable more efficient and powerful EV motors. This would also allow for the development of more compact and lightweight EV powertrains, potentially impacting the future of transportation.
Specific Demands and Requirements
Different mobile power applications have unique demands and requirements for batteries. Portable electronics prioritize factors such as form factor, weight, and power density. Electric vehicles require higher energy density, fast charging capabilities, and thermal stability to ensure safe and reliable operation at various temperatures. Solid-state batteries are expected to meet these demands, offering superior performance in various mobile power applications.
Table of Mobile Power Devices and Advantages
Mobile Power Device | Potential Advantages of Solid-State Batteries |
---|---|
Smartphones | Increased battery life, smaller form factor, faster charging, improved safety |
Laptops | Longer battery life, higher performance, smaller size, improved durability |
Electric Vehicles | Extended driving range, faster charging, higher power output, improved safety |
Portable Power Banks | Higher energy density, smaller size, improved safety, faster charging |
Wearable Electronics | Increased battery life, smaller form factor, improved safety |
Impact on Overall Efficiency, Solid-state batteries smartphone mobile power future
Solid-state batteries have the potential to significantly enhance the overall efficiency of mobile power systems. Their higher energy density translates to greater power output and reduced battery weight, potentially reducing the size of power systems required for a given application. Furthermore, improved safety and thermal stability can reduce the need for complex safety mechanisms, leading to more efficient and reliable systems.
Consequently, this could lower the overall cost and environmental impact of mobile power solutions.
Future of Solid-State Batteries
Solid-state batteries are poised to revolutionize energy storage, offering significant advantages over traditional lithium-ion batteries. Their potential impact spans from portable electronics to large-scale energy grids, presenting exciting possibilities and challenges. Early adopters are keen to see how these batteries can deliver higher energy density, improved safety, and longer lifecycles.Research and development efforts in solid-state batteries are focused on overcoming current limitations and accelerating the path to commercialization.
Key areas of advancement include material science breakthroughs, manufacturing process optimizations, and battery cell design enhancements. These efforts promise a future where solid-state batteries can meet the demanding requirements of modern electronics and beyond.
Current Research and Development Efforts
Researchers are actively exploring a variety of solid electrolytes, including ceramic materials, polymer composites, and glass-ceramics. This exploration is driven by the need for electrolytes that exhibit high ionic conductivity, excellent stability, and compatibility with lithium metal anodes. Significant advancements are being made in controlling the grain size and microstructure of solid electrolytes to enhance their performance. These advancements, in turn, contribute to improving the overall efficiency and safety of solid-state battery designs.
Projected Timelines for Commercialization
While precise timelines are difficult to predict, industry projections suggest that solid-state batteries may enter the commercial market for smartphones and other mobile power applications within the next 5-10 years. The timeline is contingent upon successful resolution of challenges related to cost-effective manufacturing, scalability, and long-term stability. The development and deployment of these technologies are likely to follow a phased approach, starting with niche applications and progressively moving towards broader adoption.
Early demonstrations and pilot programs are crucial to validating performance and building confidence among manufacturers.
Potential Impact on the Global Energy Landscape
Solid-state batteries hold the potential to significantly impact the global energy landscape. Their higher energy density and longer lifecycles could enable the development of more efficient and compact energy storage solutions for electric vehicles (EVs), grid-scale energy storage systems, and portable electronics. This, in turn, can contribute to a more sustainable energy future. Their potential to power EVs with longer ranges and faster charging times, for example, could disrupt the automotive industry and reshape transportation.
Societal Implications of Widespread Adoption
Widespread adoption of solid-state batteries could have profound societal implications. Improved energy storage solutions could lead to a reduction in reliance on fossil fuels, contributing to a lower carbon footprint. The development of more efficient and compact energy storage solutions can contribute to the development of more sustainable and efficient devices and technologies. The increased affordability and accessibility of sustainable energy solutions could lead to economic growth and development in emerging markets.
Potential Benefits and Drawbacks
Potential Benefits | Potential Drawbacks |
---|---|
Higher energy density | Higher manufacturing costs |
Improved safety | Challenges in scalability |
Longer lifespan | Limited availability of raw materials |
Reduced environmental impact (potentially) | Material incompatibility and degradation issues |
Enhanced performance in demanding applications (e.g., EVs) | Long-term reliability and stability issues |
Manufacturing and Cost Considerations: Solid-state Batteries Smartphone Mobile Power Future
Solid-state batteries, while promising, face significant hurdles in their path to widespread adoption. A crucial aspect of this journey is understanding the manufacturing processes and the associated cost implications. The complexities of production and the potential for higher costs compared to lithium-ion batteries are key factors in determining the timeline and scale of their deployment.Manufacturing solid-state batteries presents challenges not encountered in lithium-ion production.
These challenges range from the intricate materials science required for the solid electrolyte to the sophisticated techniques needed for cell assembly. Consequently, the cost structure for solid-state batteries may differ significantly, demanding careful evaluation to identify potential cost reduction strategies.
Solid-State Battery Manufacturing Processes
The production of solid-state batteries often involves several steps, each demanding specialized equipment and expertise. These steps typically include material synthesis, electrolyte processing, cathode and anode fabrication, and cell assembly. The intricate nature of these processes contributes to the higher manufacturing costs compared to lithium-ion batteries. Significant research and development efforts are focused on optimizing these processes to enhance efficiency and reduce costs.
Cost Comparison: Solid-State vs. Lithium-Ion Batteries
The manufacturing costs of solid-state batteries are anticipated to be higher than those of lithium-ion batteries, at least in the near term. This disparity stems from several factors, including the more complex materials used, the specialized equipment required, and the lower production volumes. While the long-term cost potential of solid-state batteries remains promising, significant improvements in manufacturing techniques are needed to bring costs in line with current lithium-ion technology.
Scalability Challenges in Mass Production
Mass-producing solid-state batteries faces considerable scalability challenges. The intricacies of the manufacturing process, combined with the need for specialized equipment and highly controlled environments, can hinder the rapid scaling required for widespread adoption. Developing robust and cost-effective manufacturing processes capable of handling large-scale production is critical for the long-term viability of solid-state batteries. Further research into automation and process optimization will be critical in achieving this.
Innovations in Battery Manufacturing Techniques
Several innovative techniques are being explored to reduce the cost of solid-state battery production. These include the development of novel manufacturing processes, such as 3D printing for electrode fabrication, and the optimization of material synthesis to reduce material waste and improve efficiency. The application of advanced materials science and engineering principles will be crucial in achieving these cost reductions.
Table: Manufacturing Cost Comparison
Characteristic | Solid-State Battery | Lithium-Ion Battery |
---|---|---|
Raw Material Costs | Higher, due to specialized and often rare earth elements. | Lower, using more readily available materials. |
Production Processes | More complex, demanding sophisticated equipment and precise control. | Relatively simpler, with established manufacturing procedures. |
Manufacturing Costs (per kWh) | Estimated higher (potentially 2-3 times) in the near term. | Lower, currently established costs. |
Scalability | Lower initial scalability, requiring significant investment in infrastructure. | Higher initial scalability due to existing infrastructure. |
Safety and Reliability
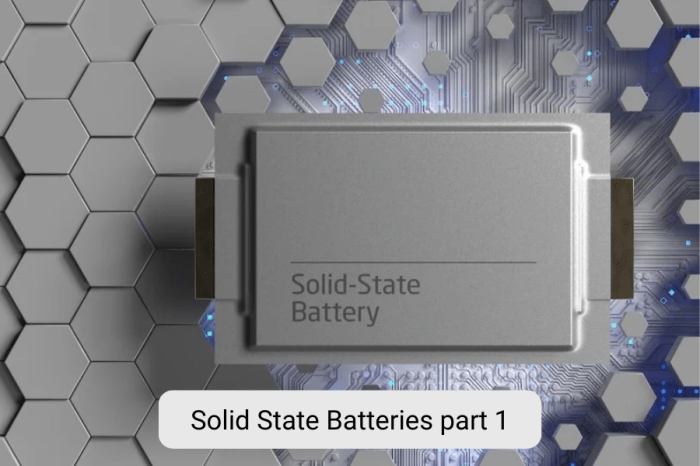
Source: ipowerbatteries.in
Solid-state batteries (SSBs) represent a promising advancement in energy storage, but their safety and reliability characteristics are critical for widespread adoption. Understanding the potential risks and implementing robust mitigation strategies are paramount to ensuring their safe integration into mobile power applications. Careful consideration of the differences between SSB and lithium-ion (Li-ion) battery chemistry is vital.The safety profile of solid-state batteries differs significantly from that of lithium-ion batteries due to the inherent nature of the solid electrolyte.
This difference necessitates a thorough evaluation of potential hazards and the development of corresponding safety protocols. Furthermore, the reliability of SSBs under various operating conditions, such as extreme temperatures and high-current discharges, must be rigorously assessed.
Safety Aspects of Solid-State Batteries
Solid-state batteries, employing a solid electrolyte instead of a liquid one, generally exhibit a reduced risk of thermal runaway compared to lithium-ion batteries. This is because the solid electrolyte inhibits the rapid propagation of thermal reactions that can lead to fires or explosions. However, other potential hazards, such as mechanical stress-induced failures or electrolyte decomposition under specific conditions, require careful attention.
Reliability Under Operating Conditions
The reliability of solid-state batteries is contingent upon the material properties of the components and the manufacturing process. Factors like temperature fluctuations, mechanical stress during operation, and the rate of charge and discharge cycles significantly impact the lifespan and performance of these batteries. Extensive testing under various conditions, including extreme temperatures and high-stress environments, is crucial to establish reliability metrics.
Potential Risks and Mitigation Strategies
Potential risks associated with solid-state batteries include, but are not limited to, mechanical degradation, electrolyte decomposition, and issues arising from interfacial interactions. Mitigation strategies often involve material selection, advanced manufacturing techniques, and robust quality control measures. Employing advanced diagnostics and monitoring systems can also help detect and prevent potential failures. For instance, implementing safety interlocks and incorporating thermal management systems can effectively mitigate risks.
Rigorous Testing and Quality Control
Rigorous testing procedures and stringent quality control measures are essential for ensuring the safety and reliability of solid-state batteries. These procedures should encompass various aspects, including material characterization, electrochemical testing, and accelerated aging tests. Independent verification of the battery’s performance and safety characteristics is critical to establish trust and confidence in the technology. Continuous monitoring and analysis of failure modes, particularly during high-stress conditions, help refine design and manufacturing processes.
Safety and Reliability Standards
Characteristic | Description | Li-ion Battery Standard | Solid-State Battery Standard (Proposed/Emerging) |
---|---|---|---|
Thermal Runaway | Likelihood of uncontrolled temperature increase | High risk; flammable electrolyte | Lower risk; solid electrolyte |
Electrolyte Safety | Safety of the electrolyte | Liquid electrolyte poses flammability risks | Solid electrolyte reduces flammability |
Mechanical Integrity | Resistance to mechanical stress | Generally robust, but can degrade over time | Developing robust design; emphasis on mechanical stability |
Electrochemical Stability | Stability during charge/discharge cycles | Good, but degradation over time | Developing standards for long-term stability |
Manufacturing Process | Quality control in production | Established standards, but ongoing improvement | Emerging standards, emphasis on process control |
This table provides a concise overview of the key differences in safety and reliability standards between Li-ion and SSB. Ongoing research and development are continuously refining these standards to meet the demands of advanced mobile power applications.
Final Conclusion
In conclusion, the future of mobile power hinges on the successful development and implementation of solid-state batteries. While challenges remain in manufacturing, cost, and safety, the potential benefits in terms of performance, efficiency, and safety are substantial. Continued research and development in this field are crucial to unlocking the full potential of solid-state batteries and their transformative impact on the future of mobile devices and beyond.
Post Comment