Quantum Computing Impact Tech World A Revolution
Introduction to Quantum Computing
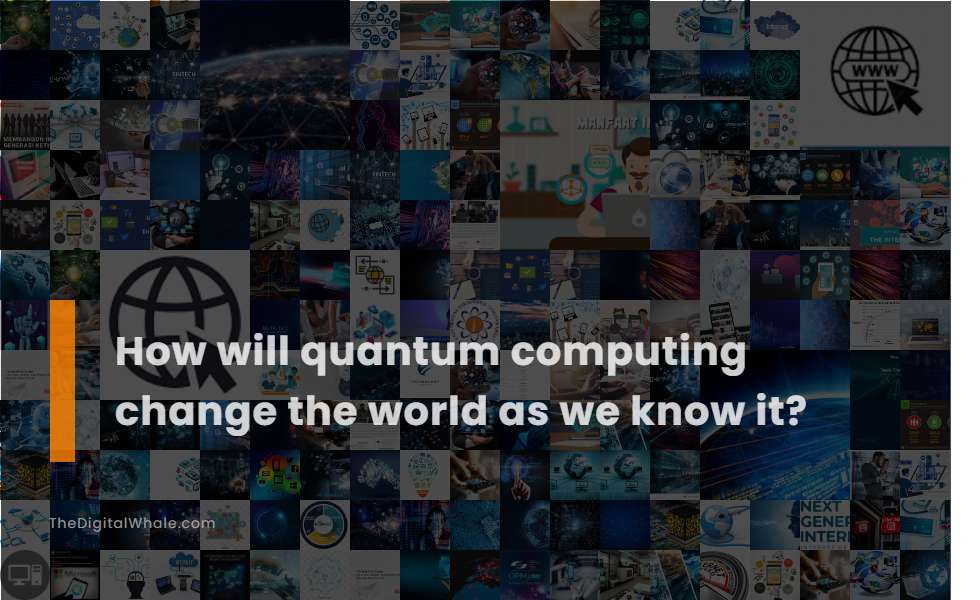
Source: thedigitalwhale.com
Quantum computing represents a paradigm shift in computation, leveraging the principles of quantum mechanics to perform calculations in ways fundamentally different from classical computers. This novel approach holds the potential to solve complex problems currently intractable for even the most powerful supercomputers, revolutionizing fields from drug discovery to materials science. Its development is a complex and exciting area of research, with significant implications for the future.
Quantum Computing Principles
Quantum computing operates on the principles of quantum mechanics, which govern the behavior of matter at the atomic and subatomic level. Unlike classical bits, which represent 0 or 1, quantum bits, or qubits, can exist in a superposition of both states simultaneously. This superposition, combined with entanglement, allows quantum computers to explore multiple possibilities simultaneously, leading to exponential speedups for certain types of calculations.
Classical vs. Quantum Computing
Classical computers rely on bits, which are binary digits representing either 0 or 1. Quantum computers, in contrast, use qubits, which can be 0, 1, or a superposition of both. This fundamental difference in the nature of information processing allows quantum computers to tackle problems that are currently beyond the reach of classical computers. The table below summarizes the key differences.
Feature | Classical Computer | Quantum Computer |
---|---|---|
Basic Unit | Bit (0 or 1) | Qubit (0, 1, or superposition of both) |
Information Processing | Sequential, one calculation at a time | Parallel, exploring multiple possibilities simultaneously |
Problem Solving | Limited to algorithms suited to sequential processing | Potentially capable of solving complex problems beyond the reach of classical computers |
Current Applications | Wide range of applications, including data processing, simulations, and more. | Emerging applications in specific problem domains. |
Current Limitations | Limited by the laws of classical physics | Limited by the challenges of qubit control and scalability |
Qubits, Superposition, and Entanglement
Qubits are the fundamental units of quantum information. They can exist in a superposition of states, meaning they can be both 0 and 1 simultaneously, represented mathematically as α|0⟩ + β|1⟩, where α and β are complex numbers. This property allows quantum computers to explore multiple possibilities concurrently. Entanglement is another crucial quantum phenomenon where two or more qubits become linked in such a way that their fates are intertwined. Even when separated by vast distances, changes to one entangled qubit instantaneously affect the other. This property is essential for certain quantum algorithms.
A key example of superposition is flipping a coin. A classical bit can only be either heads or tails, but a qubit can be both heads and tails at the same time until measured.
Strengths and Weaknesses of Quantum Computers, Quantum computing impact tech world
Quantum computers offer significant advantages over classical computers in specific problem domains, particularly in areas involving complex calculations and simulations. However, they also face significant challenges in terms of scalability and control. This table highlights the comparative strengths and weaknesses.
Feature | Classical Computer Strengths | Classical Computer Weaknesses | Quantum Computer Strengths | Quantum Computer Weaknesses |
---|---|---|---|---|
Computational Power | Excellent for many everyday tasks | Limited for complex calculations and simulations | Potentially exponential speedup for specific problems | Limited by the difficulty of building and maintaining quantum computers |
Problem Solving | Efficient for tasks with well-defined solutions | Struggles with certain problems like drug discovery and materials science | Can potentially address problems currently beyond classical computers | Developing and applying quantum algorithms remains a challenge |
Scalability | Relatively easy to scale up classical computers | Not a major concern in most classical applications | Very difficult to scale up qubits reliably | Current quantum computers are relatively small |
Current Applications in Technology
Quantum computing, despite its nascent stage, is already demonstrating potential across diverse technological sectors. Early applications leverage the unique capabilities of quantum algorithms to tackle complex problems currently intractable for classical computers. These advancements hold promise for revolutionizing fields ranging from drug discovery to financial modeling.
Early Applications in Medicine
Quantum computing’s potential in medicine lies in its ability to simulate molecular interactions with unprecedented accuracy. This allows researchers to model the behavior of molecules, including proteins and DNA, with far greater detail than classical methods. Simulating complex chemical reactions, like those involved in drug development, is a key application. Faster and more accurate simulations could significantly reduce the time and resources required to discover new drugs and treatments.
Early Applications in Materials Science
Quantum algorithms offer the potential to design and optimize materials with tailored properties. By modeling the behavior of atoms and molecules at a quantum level, researchers can predict and design materials with specific characteristics. This includes properties like strength, conductivity, and chemical reactivity. This could lead to the development of new materials for applications in electronics, energy storage, and other areas. This area is particularly promising for the creation of novel catalysts for chemical reactions, enhancing industrial processes and reducing environmental impact.
Early Applications in Finance
Quantum algorithms can potentially improve financial modeling and risk management. Quantum computers can efficiently solve optimization problems, which are crucial in financial markets. This could lead to more accurate risk assessments, better portfolio optimization, and improved fraud detection. Quantum machine learning techniques might also improve the speed and accuracy of trading algorithms, leading to more efficient and profitable financial strategies.
Quantum Algorithms in Action
Quantum algorithms are central to the applications discussed. Algorithms like Shor’s algorithm, for instance, can efficiently factor large numbers, potentially impacting cryptography. Other algorithms, like Grover’s algorithm, can accelerate search tasks, improving the efficiency of database searches and other processes. The development and implementation of these specialized quantum algorithms are crucial for harnessing the power of quantum computing.
Impact on Existing Tech Processes
Quantum computing is not replacing existing technologies, but rather complementing them. By enabling solutions to complex problems currently beyond the capabilities of classical computers, quantum computing has the potential to significantly enhance existing technological processes. This includes speeding up simulations, improving data analysis, and enabling new types of optimization.
Key Tech Sectors with Early Applications
Tech Sector | Specific Application |
---|---|
Medicine | Drug discovery, protein folding simulations |
Materials Science | Material design, catalyst optimization |
Finance | Risk assessment, portfolio optimization |
Cryptography | Breaking existing encryption methods |
Artificial Intelligence | Accelerating machine learning algorithms |
Potential Future Impacts
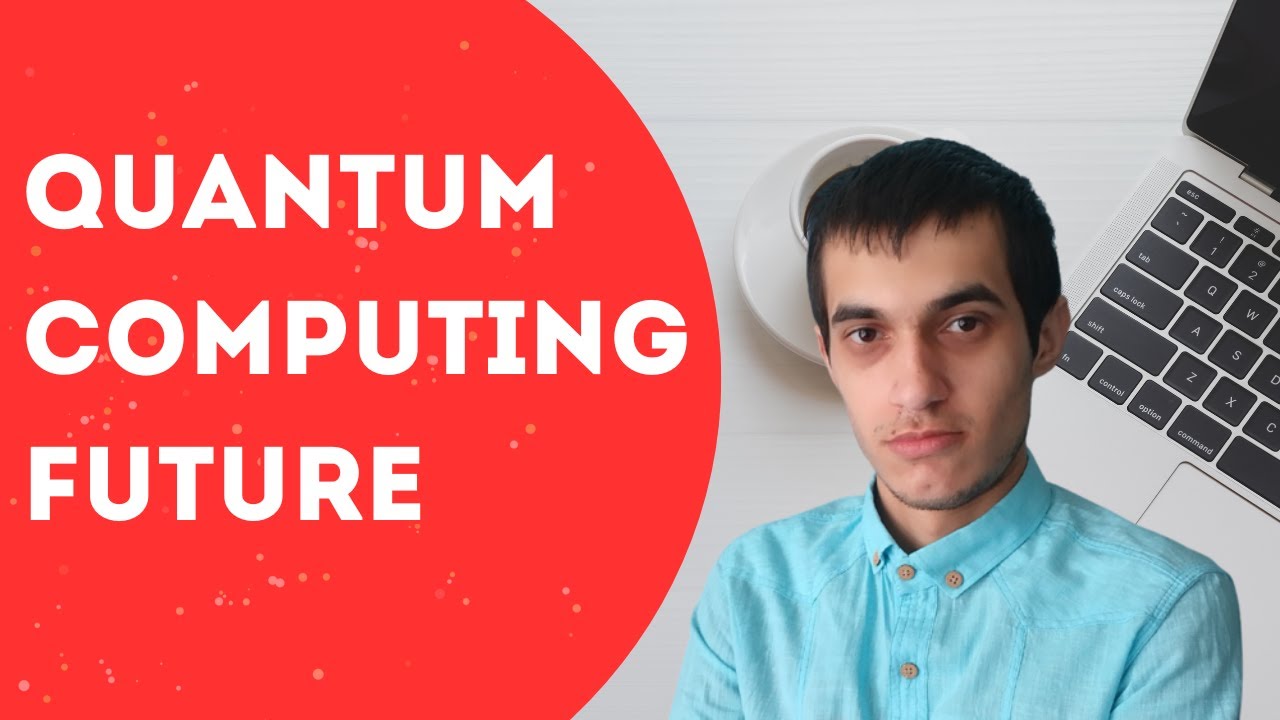
Source: ytimg.com
Quantum computing’s trajectory promises transformative changes across numerous sectors. Its potential to solve complex problems currently intractable for classical computers opens doors to breakthroughs in materials science, drug discovery, and artificial intelligence. Early adoption in specialized fields suggests a rapid acceleration in the development and application of quantum algorithms and hardware.
Advancements in Quantum Computing Technology
Quantum computing technology is rapidly evolving, driven by improvements in qubit coherence times and error correction techniques. Increased qubit counts and enhanced control over quantum systems will lead to more powerful and versatile quantum computers. This progress will allow for tackling more intricate problems and developing more sophisticated algorithms. The development of novel quantum hardware architectures, such as trapped ions, superconducting circuits, and photonic qubits, will broaden the spectrum of problems amenable to quantum solutions. Furthermore, the integration of quantum computing with existing technologies will accelerate its practical implementation.
Breakthroughs in Quantum Algorithms and Hardware
Significant breakthroughs in quantum algorithms are anticipated. The development of new algorithms tailored to specific problems, such as optimization and machine learning, will unlock new possibilities. Quantum machine learning algorithms are poised to revolutionize data analysis and pattern recognition, potentially leading to advancements in areas like drug discovery and financial modeling. Simultaneously, hardware advancements will drive improved qubit quality and control, enabling more accurate and reliable computations. Quantum error correction protocols are crucial for scaling quantum computers and mitigating the effects of noise, which are essential for practical applications.
Reshaping Existing Industries
Quantum computing has the potential to revolutionize several industries. In pharmaceuticals, quantum simulations can accelerate drug discovery and design, potentially reducing development time and costs. Materials science could benefit from simulations of complex materials, leading to new materials with enhanced properties. Financial modeling and optimization can be transformed by quantum algorithms, potentially leading to improved risk assessment and portfolio management. Furthermore, quantum computing could enhance logistics and supply chain optimization through better resource allocation and route planning.
Potential Future Impacts on Everyday Life
The impacts of quantum computing will extend beyond specialized industries. Improved drug discovery could lead to more effective and targeted medications, impacting healthcare. Enhanced materials science could lead to more sustainable and efficient products, affecting consumer goods. Advanced simulations in weather forecasting and climate modeling could improve our understanding of global environmental issues. Improved financial modeling and optimization could potentially impact personal finance and investment strategies.
Potential Future Applications Across Various Sectors
Sector | Potential Application |
---|---|
Pharmaceuticals | Accelerated drug discovery and design, personalized medicine |
Materials Science | Development of new materials with enhanced properties, sustainable manufacturing |
Finance | Improved risk assessment, portfolio optimization, fraud detection |
Logistics | Optimized supply chains, efficient route planning |
Energy | Advanced battery technology, improved energy efficiency |
Artificial Intelligence | Enhanced machine learning algorithms, improved pattern recognition |
Environmental Science | Advanced climate modeling, improved weather forecasting |
Challenges and Limitations
Quantum computing, while promising revolutionary advancements, faces significant hurdles in its widespread adoption. These challenges stem from the delicate nature of quantum systems, requiring extremely controlled environments and sophisticated engineering to maintain quantum coherence. The inherent complexity of quantum algorithms and hardware further complicates the practical implementation of these powerful technologies.
Major Challenges Hindering Widespread Adoption
The journey towards widespread quantum computing adoption is fraught with obstacles. These challenges span across several key areas, impacting everything from the physical realization of qubits to the development of robust quantum algorithms. The need for extremely low temperatures, high precision control, and error mitigation presents significant engineering hurdles. Furthermore, the current lack of standardized protocols and interoperability between different quantum computing platforms further hinders progress.
Technical Limitations of Current Quantum Computing Hardware
Current quantum computers suffer from limitations in qubit coherence time and connectivity. Qubits, the fundamental units of quantum information, are extremely susceptible to environmental noise, causing them to lose their quantum properties (decoherence). This limitation significantly restricts the duration and complexity of computations that can be performed. Furthermore, the limited connectivity between qubits can constrain the scalability and efficiency of quantum algorithms.
Need for Further Research and Development
Continued research and development are essential to address the shortcomings of current quantum computing hardware. Focus areas include improving qubit coherence times, enhancing qubit connectivity, and developing robust error correction techniques. The development of more sophisticated quantum algorithms tailored to specific applications is also crucial for realizing the full potential of quantum computing. This necessitates a collaborative effort between physicists, engineers, and computer scientists to overcome these challenges.
Cost of Implementing Quantum Computing Systems
The high cost of building and maintaining quantum computers is a significant barrier to their widespread adoption. The sophisticated infrastructure required, including cryogenic cooling systems and precise control mechanisms, is extremely expensive to develop and maintain. Furthermore, the specialized expertise required to operate and program these systems adds to the overall cost.
Challenges and Proposed Solutions in Quantum Computing Hardware
Challenge | Proposed Solution |
---|---|
Qubit coherence time | Developing novel qubit designs with improved resilience to environmental noise, employing advanced error correction techniques, and creating more stable isolation environments. |
Qubit connectivity | Designing and implementing more efficient and scalable qubit interconnection schemes, potentially leveraging advanced nanotechnology for better integration. |
Scalability | Focusing on developing modular and scalable architectures that allow for gradual expansion and integration of additional qubits. |
Error correction | Implementing robust error correction protocols to mitigate the effects of decoherence and other errors during quantum computations. Developing more fault-tolerant quantum algorithms and architectures. |
Cost | Developing more cost-effective cooling and control technologies, optimizing manufacturing processes, and exploring alternative materials for qubit construction. |
The Quantum Computing Ecosystem

Source: artofpro.com
The quantum computing landscape is rapidly evolving, with a mix of established tech giants and innovative startups vying for a piece of the action. Funding is crucial for research and development, while collaborations between these players are shaping the future of the field. Government policies play a vital role in supporting this emerging technology.
Current Landscape of Quantum Computing Companies and Research Institutions
The quantum computing ecosystem encompasses a diverse range of players, from established tech companies like Google and IBM to specialized startups developing unique hardware and software. Research institutions, both academic and government-funded, are also actively contributing to the field through cutting-edge research and development. These institutions often collaborate with industry players, fostering a synergistic environment for innovation. Many companies are focusing on specific aspects of quantum computing, such as specialized hardware or particular software algorithms, creating a competitive and dynamic market.
Funding and Investment Trends
Investment in quantum computing is experiencing significant growth. Venture capital firms are actively seeking opportunities in this promising field, recognizing its potential to revolutionize various sectors. Large corporations, with their extensive resources, are also increasingly investing in quantum computing initiatives, driven by the prospect of gaining a competitive edge in the future. This funding fuels the development of new technologies and fosters the creation of specialized quantum computing centers. Estimates suggest that venture capital funding in quantum computing will continue to grow, reflecting the increasing confidence in the technology’s future.
Collaborations and Partnerships
Strategic partnerships between tech giants and quantum computing startups are common. This collaboration often involves knowledge sharing, technology transfer, and joint research efforts. Tech giants leverage startups’ innovative ideas, while startups gain access to resources and market reach through partnerships. For example, IBM has established numerous partnerships with academic institutions and startups to accelerate research and development. This collaboration is crucial for fostering the necessary synergy to bring quantum computing solutions to market.
Government Support and Policies
Governments worldwide are recognizing the strategic importance of quantum computing and are implementing supportive policies. These policies aim to stimulate research and development, attract investment, and foster talent development in this emerging field. Government funding for quantum computing research and development initiatives is increasing in many countries, with incentives for both academic institutions and private companies. Such policies are critical for ensuring the long-term viability and growth of the quantum computing ecosystem.
Key Players in the Quantum Computing Ecosystem
Company/Institution | Role |
---|---|
IBM | Leading quantum computing hardware and software provider, significant research investments. |
Significant quantum computing research efforts, focusing on hardware development and applications. | |
Microsoft | Developing quantum computing software tools and platforms, aiming for wider accessibility. |
Rigetti Computing | Developing superconducting quantum processors, focusing on specialized hardware solutions. |
IonQ | Developing trapped-ion quantum computers, known for their specific approach to quantum hardware. |
National Institutes of Standards and Technology (NIST) | Conducts quantum computing research and development, contributes to standards and guidelines. |
University of California, Berkeley | Active research on quantum computing, strong theoretical and experimental research programs. |
Quantum Computing and Society: Quantum Computing Impact Tech World
Quantum computing, while promising transformative power, presents intricate ethical and societal challenges. Its potential to revolutionize industries necessitates careful consideration of its broader impact on society, including the workforce, and the ethical implications of its deployment. The speed and scale of quantum algorithms’ potential impact demand proactive measures to mitigate risks and maximize benefits.
The rapid advancement of quantum computing raises critical questions about its societal impact. From its potential to accelerate scientific discoveries to its possible role in exacerbating existing inequalities, a nuanced understanding is crucial. Careful consideration of the potential implications for various sectors is essential to navigating this transformative technology responsibly.
Ethical Considerations
The development and deployment of quantum computing raise numerous ethical concerns. These range from issues of access and equity to the potential misuse of the technology for malicious purposes. Ensuring responsible development and deployment is paramount to harnessing the benefits while mitigating potential harms. Security vulnerabilities in quantum algorithms and infrastructure, for instance, could have significant implications for data privacy and national security.
Societal Implications of Breakthroughs
Quantum computing breakthroughs could trigger profound societal shifts. The potential for accelerated scientific discoveries could lead to breakthroughs in medicine, materials science, and energy production. However, these advancements also carry potential risks. For instance, the creation of novel materials could disrupt existing industries and create new economic disparities. These potential changes demand careful consideration and proactive strategies for adaptation.
Impact on Jobs and the Workforce
The emergence of quantum computing will inevitably impact the workforce. While some jobs may become obsolete due to automation, new roles will emerge requiring specialized skills in quantum computing. The need for skilled personnel to develop, implement, and maintain quantum systems will likely increase. Furthermore, retraining programs and educational initiatives will be essential to ensure a smooth transition for workers.
Ethical Concerns and Potential Solutions
Ethical Concern | Potential Solution |
---|---|
Access and Equity: Unequal access to quantum resources and expertise could exacerbate existing societal disparities. | Promoting open-source development and collaborative initiatives to broaden access and democratize quantum computing technology. Funding programs targeting underrepresented groups and developing educational programs. |
Misuse of the technology: Quantum algorithms could be exploited for malicious purposes, like breaking current encryption methods. | Developing robust quantum-resistant encryption protocols and working with international collaborations to establish ethical guidelines for the development and deployment of quantum technologies. |
Job displacement: Automation driven by quantum algorithms could lead to job losses in certain sectors. | Investing in retraining programs and educational initiatives to equip workers with the skills necessary for emerging quantum-related jobs. Developing strategies to adapt and transition the workforce. |
Environmental impact: The energy consumption of quantum computers may pose a significant environmental challenge. | Prioritizing energy-efficient quantum computing architectures and focusing research on reducing the environmental footprint of these technologies. |
Quantum Computing and Cybersecurity
Quantum computing, while promising revolutionary advancements, poses a significant threat to current cybersecurity infrastructure. The inherent power of quantum algorithms could render many existing encryption methods obsolete, potentially jeopardizing sensitive data and communication channels. This necessitates a proactive approach to develop and implement quantum-resistant cryptography.
Impact on Existing Encryption
Quantum computers, with their ability to perform calculations exponentially faster than classical computers, could potentially break widely used encryption methods, such as RSA and ECC. These algorithms rely on the difficulty of factoring large numbers or solving discrete logarithm problems, tasks that become trivial for quantum computers employing Shor’s algorithm. This vulnerability affects various sectors, including finance, healthcare, and government, where data encryption is crucial for maintaining confidentiality and integrity. For example, if a quantum computer capable of breaking current encryption methods were to exist, financial transactions and sensitive medical records could be exposed to unauthorized access.
Quantum-Resistant Cryptography
The development of quantum-resistant cryptography is paramount to mitigating the threat posed by quantum computers. These new cryptographic techniques are designed to withstand attacks from quantum computers, employing mathematical problems that are intractable for both classical and quantum computers. This proactive measure is crucial to ensuring the continued security of digital assets in a post-quantum world. Post-quantum cryptography (PQC) research focuses on algorithms that resist attacks from quantum computers.
Efforts in Counteracting the Threat
Various organizations and researchers are actively engaged in developing and standardizing quantum-resistant cryptographic algorithms. International standards organizations, like NIST, are leading efforts in selecting and validating quantum-resistant algorithms, ensuring a common framework for future security. This process involves rigorous testing and analysis to identify robust and efficient alternatives to existing encryption methods. For example, NIST is currently working to standardize post-quantum cryptography algorithms, which will ensure a standardized approach to secure communications.
Vulnerabilities and Proposed Solutions in Cybersecurity
Vulnerability | Proposed Solution |
---|---|
Existing encryption methods (RSA, ECC) vulnerable to Shor’s algorithm | Development and standardization of quantum-resistant cryptographic algorithms (e.g., lattice-based cryptography, code-based cryptography, multivariate cryptography). |
Potential for large-scale data breaches | Implementation of quantum-resistant cryptography in critical infrastructure, financial systems, and healthcare sectors. Investing in research and development to keep pace with evolving quantum computing capabilities. |
Dependence on outdated encryption standards | Proactive migration to quantum-resistant cryptography, phased rollout plans for businesses and organizations. Public awareness campaigns and education programs to inform users about the risks and the need for adaptation. |
Quantum Computing and Artificial Intelligence
Quantum computing holds the potential to revolutionize artificial intelligence (AI), enabling the development of more powerful and sophisticated AI systems. By leveraging the unique properties of quantum mechanics, these systems could address problems currently intractable for classical computers, paving the way for breakthroughs in various fields. The synergy between these two rapidly evolving technologies promises to accelerate advancements in AI and related fields.
Quantum computing’s ability to explore vast solution spaces concurrently offers a significant advantage over classical computing methods. This capability is particularly relevant in AI, where training machine learning models often requires extensive computation and exploration of numerous possibilities.
Quantum Acceleration of Machine Learning Tasks
Quantum algorithms can significantly accelerate certain machine learning tasks. Quantum computers can perform operations on many data points simultaneously, potentially leading to substantial speedups in tasks like training neural networks and clustering. This concurrent processing is a key differentiator, enabling the handling of massive datasets in a fraction of the time required by classical computers.
Quantum Machine Learning for Complex Problems
Quantum machine learning algorithms have the potential to address complex problems that are beyond the capabilities of classical machine learning approaches. These problems include: optimizing intricate systems, modeling complex phenomena, and developing more accurate predictions in areas like drug discovery, material science, and financial modeling. Quantum machine learning could lead to breakthroughs in these fields, yielding more precise models and faster solutions.
Quantum Machine Learning and AI Synergies
Quantum computing and AI are deeply intertwined, creating numerous synergistic opportunities. For example, quantum algorithms can be designed to solve specific AI problems, accelerating tasks like feature extraction and pattern recognition. This collaboration will lead to more sophisticated AI systems capable of learning and adapting more efficiently. The combination of quantum computing’s speed and AI’s ability to learn and adapt could lead to powerful and innovative solutions to complex problems.
Classical vs. Quantum Machine Learning
Feature | Classical Machine Learning | Quantum Machine Learning |
---|---|---|
Data Representation | Data is represented as vectors or matrices in a classical vector space. | Data can be represented in a quantum state, allowing for more efficient encoding and processing. |
Computation | Computation is performed sequentially on individual data points. | Computation can be performed on multiple data points simultaneously, leading to potentially exponential speedups. |
Algorithms | Algorithms like linear regression, support vector machines, and neural networks. | Algorithms like quantum support vector machines, quantum neural networks, and variational quantum algorithms. |
Data Size Limitations | Scalability can be limited by the size of the data. | Potentially better scalability for handling large datasets. |
Current State | Widely used and mature technologies. | Still under development and research. |
Quantum machine learning, though still in its early stages, is a rapidly evolving field with immense potential. This comparison illustrates the fundamental differences in how classical and quantum approaches handle data and computation, highlighting the potential for quantum computing to enhance AI capabilities.
Post Comment