History Of Wi-Fi Technology And Its Workings
History of Wi-Fi technology and its workings explores the fascinating evolution of wireless communication. From the early days of radio waves to the sophisticated standards of today, this journey unveils the key innovations that shaped Wi-Fi. We’ll delve into the underlying technologies, examine the different frequency bands, and uncover the intricacies of network architecture. The discussion further extends to security protocols, modern applications, and the exciting future trends in Wi-Fi.
This exploration traces the path from basic wireless concepts to the intricate details of Wi-Fi’s inner workings. It demonstrates how advancements in modulation, encoding, and security protocols have enabled Wi-Fi to become the ubiquitous technology it is today. Furthermore, the analysis highlights the importance of Wi-Fi in contemporary applications, ranging from IoT devices to high-speed internet access.
Early Days of Wireless Communication
The quest for wireless communication predates Wi-Fi by many decades. Early pioneers experimented with various methods to transmit signals without wires, paving the way for the technologies we use today. These early efforts, though sometimes rudimentary, laid the foundation for the sophisticated wireless networks we rely on.Wireless communication, in its nascent stages, relied on technologies that were limited in range, bandwidth, and reliability.
These early approaches often struggled to transmit data effectively and efficiently, necessitating substantial improvements before the development of Wi-Fi. These early technologies, while not as versatile as Wi-Fi, were crucial stepping stones in the evolution of wireless communication.
Historical Overview of Preceding Wireless Technologies
Early wireless communication technologies, like radio telegraphy and early radio broadcasting, utilized electromagnetic waves to transmit information. These systems, while capable of transmitting signals over considerable distances, were primarily employed for voice and simple data transmission. Key examples include Morse code transmission and early radio broadcasts, which often suffered from interference and limited bandwidth.
Key Challenges and Limitations of Early Wireless Technologies
These early wireless systems faced numerous challenges. Limited bandwidth meant that only a small amount of data could be transmitted at a time, making them unsuitable for high-speed applications. Signal interference from other sources was also a major problem, leading to signal degradation and data loss. The lack of standardization between different systems also posed a significant obstacle, preventing seamless communication between various devices.
Furthermore, power limitations and range constraints restricted the utility of these systems.
Motivations Behind the Development of Wi-Fi
The development of Wi-Fi stemmed from the need for a more versatile and efficient wireless technology. The limitations of existing wireless communication technologies, particularly in terms of speed, range, and bandwidth, fueled the desire for a more robust and reliable alternative. The growing demand for high-speed data transmission in homes and offices, along with the need for a flexible and scalable network infrastructure, drove the development of Wi-Fi.
Early Wi-Fi implementations focused on addressing these limitations, aiming for faster data transfer rates and improved connectivity.
Comparison of Wireless Communication Standards, History of Wi-Fi technology and its workings
The following table provides a comparison between various wireless communication standards preceding Wi-Fi and Wi-Fi itself, highlighting key differences and similarities.
Standard | Frequency Band | Data Rate (approx.) | Range | Applications | Limitations |
---|---|---|---|---|---|
Early Radio Telegraphy | Various, often low frequency | Low (Morse code) | Relatively long | Early communication, ship-to-shore | Very low data rate, limited functionality |
Early Radio Broadcasting | Various, often medium frequency | Low to medium | Moderate | Broadcasting of audio signals | Low data rate, prone to interference |
Wi-Fi (802.11) | 2.4 GHz and 5 GHz | Up to Gigabit speeds | Variable, typically several tens of meters indoors | Internet access, data transfer, networking devices | Susceptible to interference from other devices operating in same frequency bands. Security concerns need careful implementation. |
The table illustrates the progression from low-bandwidth, limited-functionality technologies to the more advanced and versatile Wi-Fi standard. Each generation of wireless technology built upon the strengths of previous iterations, while addressing their limitations to enhance overall performance and functionality.
Evolution of Wi-Fi Standards
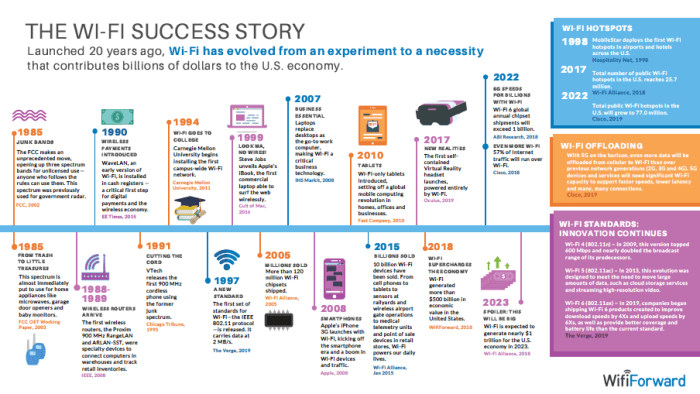
Source: wififorward.org
The evolution of Wi-Fi standards reflects a continuous quest for faster speeds, greater range, and enhanced reliability. Early standards laid the groundwork, but subsequent iterations significantly improved performance and expanded capabilities. This evolution is critical to the ubiquitous use of wireless networks today.The progression of Wi-Fi standards has been marked by increasing data transfer rates and improved efficiency.
Each new standard builds upon the strengths of its predecessors, addressing limitations and pushing the boundaries of wireless communication. Understanding these advancements is crucial to appreciating the current state of Wi-Fi and anticipating future developments.
Wi-Fi 802.11b
This standard introduced the use of the 2.4 GHz frequency band, enabling wider compatibility and broader reach compared to previous methods. 802.11b significantly improved upon earlier wireless technologies by offering a more practical data transfer rate for home and small office networks. Its maximum theoretical data transfer rate was 11 Mbps, a substantial increase over earlier standards. However, this speed was not consistently achieved in real-world scenarios.
Wi-Fi 802.11a
Operating in the 5 GHz frequency band, 802.11a boasted higher theoretical maximum speeds than 802.11b. It offered a maximum theoretical data rate of 54 Mbps, a considerable leap forward. The higher frequency band meant a smaller range compared to 802.11b, and it was less common in early adopters.
Wi-Fi 802.11g
Recognizing the need for broader compatibility, 802.11g combined the benefits of both 802.11a and 802.11b. It operated in the 2.4 GHz frequency band, ensuring compatibility with existing 802.11b devices, while achieving higher data transfer rates. Its theoretical maximum speed was 54 Mbps, making it a popular choice for home networks and small businesses.
Wi-Fi 802.11n
11n represented a significant advancement, incorporating MIMO (Multiple-Input and Multiple-Output) antenna technology. This technology enabled simultaneous data transmission on multiple antennas, substantially increasing both the speed and range of Wi-Fi. Its maximum theoretical data rate was 600 Mbps, and it supported both 2.4 GHz and 5 GHz frequency bands, allowing for more flexibility.
Wi-Fi 802.11ac
11ac capitalized on the 5 GHz frequency band, leveraging wider channels for significantly higher speeds. It utilized advanced modulation schemes and beamforming technology for improved signal strength and efficiency. The maximum theoretical data rate was 1.3 Gbps, making it ideal for high-bandwidth applications like streaming and large file transfers.
Wi-Fi 802.11ax
The latest Wi-Fi standard, 802.11ax, further improved performance by incorporating features like OFDMA (Orthogonal Frequency-Division Multiple Access), 1024-QAM modulation, and target wake time. These features enabled more efficient data transmission, allowing multiple devices to share the network simultaneously. The theoretical maximum data rate for 802.11ax was 10 Gbps. This is crucial for today’s data-intensive activities.
Comparison of Wi-Fi Standards
The following table summarizes the evolution of Wi-Fi standards, their speeds, and frequency bands:
Standard | Frequency Band(s) | Maximum Theoretical Speed (Mbps) | Key Improvements |
---|---|---|---|
802.11b | 2.4 GHz | 11 | Introduced 2.4 GHz band; increased speed over previous standards. |
802.11a | 5 GHz | 54 | Higher speeds; 5 GHz band. |
802.11g | 2.4 GHz | 54 | Combined high speed with 802.11b compatibility. |
802.11n | 2.4 GHz and 5 GHz | 600 | MIMO technology; increased speed and range. |
802.11ac | 5 GHz | 1300 | Wider channels, advanced modulation; higher speeds. |
802.11ax | 2.4 GHz and 5 GHz | 1024 | OFDMA, 1024-QAM; more efficient data transmission. |
Wi-Fi Frequency Bands and Channels
Wi-Fi networks utilize radio waves to transmit data. These waves operate within specific frequency bands, each with its own characteristics affecting performance and potential interference. Understanding these bands and their associated channels is crucial for optimal Wi-Fi network setup and troubleshooting.
Frequency Bands
Wi-Fi primarily operates in two frequency bands: 2.4 GHz and 5 GHz. These bands offer different strengths and weaknesses, impacting speed, range, and potential interference. Choosing the appropriate band depends on the specific needs of the network.
2.4 GHz Band
The 2.4 GHz band is the older of the two, offering wider coverage but with reduced speed and increased interference possibilities. Its wider range is often beneficial for larger homes or areas with obstacles.
- Advantages: Greater range compared to the 5 GHz band, and often better penetration through walls and other obstacles. More devices are compatible with 2.4 GHz.
- Disadvantages: Lower speeds compared to the 5 GHz band, and more susceptible to interference from other devices using the same frequency band (e.g., microwaves, cordless phones). More crowded with other networks.
- Common Channels: The 2.4 GHz band typically utilizes 1 to 11 channels. Channel 1, 6, and 11 are the most commonly recommended as they are spaced further apart, minimizing interference between adjacent channels.
5 GHz Band
The 5 GHz band provides higher speeds and less interference than the 2.4 GHz band, but its range is generally shorter. This band is becoming increasingly popular as more devices adopt it, leading to a more consistent connection in areas with less interference.
- Advantages: Significantly higher speeds compared to 2.4 GHz, due to the wider bandwidth available. Fewer devices operate on this frequency, leading to less interference.
- Disadvantages: Shorter range than the 2.4 GHz band. Signal penetration through walls and other obstacles can be weaker.
- Common Channels: The 5 GHz band offers many more channels, generally from 36 to 165. Different channel selections are available depending on the Wi-Fi standard.
Channel Assignments and Interference
Choosing the correct channels within each band is essential to minimize interference. Adjacent channels can overlap, causing reduced throughput and connection stability. Utilizing non-overlapping channels is crucial for maintaining strong signal quality.
Frequency Band | Channels | Potential Interference |
---|---|---|
2.4 GHz | 1, 6, 11 | Least interference from adjacent channels. |
2.4 GHz | 3, 5, 7, 9 | Can experience interference from adjacent channels, potentially impacting network performance. |
5 GHz | 36, 40, 44, 48, 149, 153, 157, 161, 165 | Fewer potential sources of interference compared to 2.4 GHz, but proper channel selection is still important. |
Wi-Fi Modulation and Encoding Techniques
Wi-Fi’s ability to deliver high data rates and reliable connections relies heavily on the modulation and encoding techniques employed. These methods translate digital data into signals that can be transmitted over the air and then accurately reconstructed at the receiving end. Understanding these techniques is crucial for appreciating the evolution of Wi-Fi standards and their increasing capacity.Modulation techniques are the cornerstone of modern Wi-Fi’s performance.
They determine how effectively data is encoded onto the radio waves and how robust the transmission is against interference. Different techniques offer varying trade-offs in terms of speed, reliability, and complexity.
Orthogonal Frequency-Division Multiplexing (OFDM)
OFDM is a key modulation technique in modern Wi-Fi standards. It divides the available radio frequency spectrum into multiple smaller, non-overlapping channels. Data is then transmitted simultaneously over these channels, significantly increasing the overall data rate. This allows for a high level of data transmission efficiency.
Quadrature Amplitude Modulation (QAM)
QAM is another vital technique used in conjunction with OFDM. It increases the data rate by increasing the number of possible signal levels that can be transmitted on each frequency channel. Higher-order QAM, such as 256-QAM or 1024-QAM, allows for more data to be encoded in each symbol, leading to faster data transfer rates.
Impact on Data Transfer Rates and Reliability
The choice of modulation and encoding techniques directly affects data transfer rates. Higher-order QAM, combined with OFDM, allows for much faster data rates compared to older techniques. However, higher-order modulation schemes are more susceptible to noise and interference, potentially impacting the reliability of the connection. Sophisticated error correction codes are employed to mitigate these effects.
Comparison Across Wi-Fi Standards
Different Wi-Fi standards utilize varying combinations of modulation and encoding schemes. Early standards often relied on simpler techniques, leading to lower data rates. Later standards, such as 802.11n and 802.11ac, incorporated more advanced schemes like OFDM and higher-order QAM, resulting in significant increases in throughput. For example, 802.11ax (Wi-Fi 6) builds on this by further optimizing OFDM and using even higher QAM orders for maximum data rates.
Impact on Wi-Fi Network Capacity
The choice of modulation techniques profoundly influences the capacity of a Wi-Fi network. More efficient modulation and encoding schemes allow more data to be transmitted over the same bandwidth. This results in increased network capacity, supporting more devices and higher data loads simultaneously. For instance, a network using 1024-QAM and OFDM can support more users and devices compared to a network employing older, less efficient techniques.
Wi-Fi Network Architecture
Wi-Fi networks, enabling wireless communication, rely on a specific architecture. This structure dictates how devices connect and interact to share data. Understanding the fundamental components and topologies is crucial for effective network design and troubleshooting. A well-designed Wi-Fi network ensures reliable and efficient data transmission among connected devices.
Fundamental Components of a Wi-Fi Network
The core components of a Wi-Fi network are access points, clients, and routers. Access points (APs) serve as the central hubs for wireless communication, acting as bridges between wireless clients and the wired network infrastructure. Clients, including laptops, smartphones, and tablets, connect wirelessly to the APs to access the network. Routers typically combine wired and wireless networking functions, allowing multiple devices to connect and share internet access through a single connection.
Network Topologies Supported by Wi-Fi
Wi-Fi supports various network topologies, each with its own characteristics and applications. These topologies define how devices are interconnected and how data flows through the network. The choice of topology depends on the specific needs and constraints of the deployment environment.
- Infrastructure Mode: In this mode, clients communicate with the network through an access point. This is the most common topology for home and office Wi-Fi networks. The access point acts as a central hub for managing and routing traffic between clients and the wired network.
- Ad-hoc Mode: In this topology, devices communicate directly with each other without an intermediary access point. This mode is often used for temporary networks or peer-to-peer file sharing between devices. Ad-hoc mode is less common in large-scale deployments because of its limited scalability and security considerations.
Communication Protocols and Processes in a Wi-Fi Network
Wi-Fi networks utilize standardized protocols and processes to enable wireless communication. These protocols ensure reliable and efficient data transmission. The protocols handle tasks like data encoding, transmission, and reception, ensuring consistent performance.
- IEEE 802.11 Standards: These standards define the communication protocols used in Wi-Fi networks, specifying details such as data rates, security measures, and channel access methods. These standards are regularly updated to accommodate the evolving needs of Wi-Fi technology.
- Media Access Control (MAC): The MAC protocol determines how devices access the shared wireless medium (the airwaves). It employs mechanisms to prevent collisions and ensure orderly transmission of data among various clients connected to the same access point. Collision avoidance and control are crucial for reliable communication in a shared wireless environment.
Different Wi-Fi Network Topologies and their Characteristics
The table below summarizes the key characteristics of the common Wi-Fi network topologies.
Topology | Description | Characteristics | Applications |
---|---|---|---|
Infrastructure Mode | Clients connect to the network through an access point. | Centralized management, scalability, security features. | Home networks, office networks, public hotspots. |
Ad-hoc Mode | Devices communicate directly with each other without an access point. | Simple setup, no central management. Limited scalability. | Temporary networks, peer-to-peer file sharing. |
Security Protocols in Wi-Fi
Wi-Fi networks, while offering convenience and flexibility, are susceptible to security breaches. Robust security protocols are crucial to protect sensitive data transmitted over these networks. This section delves into the evolution of Wi-Fi security, highlighting the key protocols, their vulnerabilities, and best practices for secure network implementation.
Security Protocols Overview
Various security protocols have been developed to enhance the confidentiality, integrity, and availability of Wi-Fi communications. Early protocols, though effective for their time, were superseded by more advanced and secure mechanisms. These protocols form a layered defense against unauthorized access and data manipulation.
WPA and WPA2 Protocols
The Wi-Fi Protected Access (WPA) protocol, introduced in 2003, addressed some of the vulnerabilities of the older Wired Equivalent Privacy (WEP) protocol. WPA utilized Temporal Key Integrity Protocol (TKIP) for encryption, offering enhanced security over WEP. WPA2, released in 2004, further strengthened security by incorporating the Advanced Encryption Standard (AES) cipher. AES provided a significantly more robust encryption mechanism than TKIP, making it resistant to a wider range of attacks.
WPA3 Protocol
WPA3, introduced in 2018, builds upon the foundation laid by WPA2. It addresses vulnerabilities in WPA2, offering enhanced protection against brute-force attacks, and improving the overall security posture of Wi-Fi networks. WPA3 introduces individualized encryption for every device on the network, known as Simultaneous Authentication of Equals (SAE), which helps secure open networks against certain types of attacks.
WPA3 also enhances password management by introducing more robust password hashing techniques.
Wi-Fi Security Vulnerabilities and Threats
Wi-Fi networks are vulnerable to various attacks, including:
- Password Cracking: Weak or easily guessable passwords can be exploited by attackers to gain unauthorized access to the network.
- Man-in-the-Middle Attacks: Attackers intercept communication between devices on the network, potentially eavesdropping on sensitive data or modifying transmitted information.
- Rogue Access Points: Unauthorized access points can be set up to intercept network traffic or gain access to sensitive data.
- Denial-of-Service Attacks: These attacks overload the network resources, preventing legitimate users from accessing the network.
- Exploiting Weaknesses in Security Protocols: Vulnerabilities in the implementation or design of security protocols can be exploited by sophisticated attackers.
Security Best Practices
Implementing strong security practices can significantly reduce the risk of Wi-Fi network breaches. These include:
- Strong Passwords: Use strong, unique passwords for Wi-Fi networks and avoid common or easily guessable passwords.
- Enable WPA3: Upgrade to WPA3 security protocols to benefit from enhanced encryption and security features.
- Regular Updates: Keep the router’s firmware updated to patch security vulnerabilities.
- Network Segmentation: Divide the network into smaller segments to limit the impact of a potential breach.
- Restrict Access: Only allow authorized devices to connect to the network.
Security Protocols Summary Table
Protocol | Strengths | Weaknesses |
---|---|---|
WEP | Basic wireless security | Highly vulnerable to cracking; weak encryption |
WPA | Improved security over WEP; TKIP encryption | Still vulnerable to some attacks; less robust than WPA2 |
WPA2 | Robust AES encryption; strong security | Vulnerable to certain attacks like KRACK; less robust than WPA3 for some use cases |
WPA3 | Enhanced security features; Individualized encryption (SAE); strong password hashing | Requires updated hardware; might be more complex to implement in some environments |
Wi-Fi in Modern Applications
Wi-Fi has transitioned from a niche technology to a ubiquitous component of modern life. Its versatility and adaptability have fueled its integration into a diverse range of applications, impacting everything from personal devices to industrial processes. This evolution has brought about both significant opportunities and unique challenges.The pervasive nature of Wi-Fi in modern applications is largely driven by its ability to provide wireless connectivity for a wide array of devices and systems.
From smart homes and personal mobile devices to Internet of Things (IoT) deployments, Wi-Fi’s role has become indispensable. This integration has led to the development of innovative applications that improve efficiency, convenience, and user experience.
Ubiquitous Use in IoT Devices
The Internet of Things (IoT) is heavily reliant on wireless communication protocols, with Wi-Fi playing a crucial role. The growing number of interconnected devices, from smart appliances to industrial sensors, necessitates a reliable and scalable network infrastructure. Wi-Fi’s established standards and widespread adoption make it a suitable choice for many IoT deployments.
Integration in Smart Homes
Smart home ecosystems heavily leverage Wi-Fi for seamless connectivity among various devices. This connectivity enables automated control of lighting, security systems, thermostats, and entertainment systems, creating a more convenient and energy-efficient living environment. The increasing complexity of smart home systems necessitates robust Wi-Fi networks to handle the growing number of connected devices.
Mobile Device Connectivity
The continued dominance of mobile devices hinges on the reliable and ubiquitous nature of Wi-Fi. From smartphones and tablets to laptops, Wi-Fi enables seamless access to the internet and other network resources. The importance of fast and stable Wi-Fi connections is paramount for optimal performance and user experience.
Detailed Explanation: Wi-Fi in Smart Homes
Smart homes rely on Wi-Fi to facilitate the interaction between various devices and systems. A typical smart home setup might include smart thermostats, lighting systems, security cameras, and smart appliances. These devices communicate with each other and with central hubs or cloud-based platforms through Wi-Fi. The Wi-Fi network acts as a communication backbone, enabling automation, remote control, and data sharing.
For instance, a smart thermostat can adjust the temperature based on the user’s schedule and environmental conditions, all facilitated by the Wi-Fi connection. This interaction between devices contributes to energy efficiency, convenience, and security within the home environment.
Challenges in Deployment
Deploying Wi-Fi in diverse applications presents several challenges. Interference from other wireless signals, limited bandwidth in certain environments, and the need for secure communication are among the key obstacles. Addressing these challenges requires innovative solutions, such as the development of advanced modulation schemes, the use of multiple frequency bands, and robust security protocols. Furthermore, managing a large number of connected devices in a smart home environment can present unique challenges in terms of network congestion and device management.
Innovative Applications and Impact
Several innovative applications demonstrate the transformative potential of Wi-Fi. Smart agriculture utilizes Wi-Fi to monitor crop conditions and optimize irrigation schedules, leading to increased yields and reduced resource consumption. Healthcare applications use Wi-Fi for remote patient monitoring and medical device integration, improving patient care and enabling more efficient healthcare delivery. The integration of Wi-Fi into various fields showcases its crucial role in modernizing numerous sectors.
Future Trends in Wi-Fi Technology: History Of Wi-Fi Technology And Its Workings
Wi-Fi technology continues its relentless evolution, driven by the ever-increasing demand for faster speeds, wider coverage, and enhanced reliability. Emerging trends and advancements promise to reshape the landscape of wireless connectivity, impacting various industries in profound ways. This section delves into the key future directions of Wi-Fi, examining the implications for diverse sectors.The relentless pursuit of faster speeds, more reliable connections, and enhanced security continues to shape the future of Wi-Fi.
This necessitates the adoption of innovative technologies and standards, driving further innovation and development. Understanding these trends is crucial for anticipating the transformative impact on various sectors and for strategic planning.
Wi-Fi 6E and Beyond
The introduction of Wi-Fi 6E, utilizing the 6 GHz frequency band, represents a significant leap forward. This new band offers significantly more available channels, leading to improved performance and reduced congestion. The wider bandwidth enables simultaneous data transmission for multiple devices, resulting in increased throughput and reduced latency. This translates to faster speeds and a smoother user experience in demanding applications, such as virtual reality (VR), augmented reality (AR), and high-definition video streaming.
Furthermore, the increased spectrum allows for higher device density without compromising performance. This is particularly crucial in crowded environments like homes, offices, and public spaces.
Mesh Networking and Scalability
Mesh networks are gaining prominence as a way to expand Wi-Fi coverage in large areas. This approach involves multiple Wi-Fi access points working together to form a seamless network. Each access point acts as a repeater, effectively extending the range and coverage area. This distributed architecture is highly scalable, allowing for effortless expansion and adaptation to changing needs.
The seamless handoff between access points ensures a consistent connection, even as users move around the network. This is particularly beneficial for large homes, businesses, and public venues.
Enhanced Security Protocols
Security is paramount in today’s connected world, and Wi-Fi is no exception. Future Wi-Fi standards are anticipated to incorporate enhanced security protocols, addressing vulnerabilities and ensuring data integrity. These improvements aim to protect against unauthorized access and malicious attacks, thereby building trust and confidence in the reliability of wireless networks. This increased security is vital for sensitive data transmission and maintaining the privacy of users.
Examples include more robust encryption mechanisms and advanced authentication methods.
Integration with Other Technologies
Future Wi-Fi standards are expected to integrate seamlessly with other technologies, such as cellular networks and satellite communications. This integration could offer enhanced coverage, particularly in areas with limited Wi-Fi infrastructure. This combined approach aims to provide a ubiquitous, high-speed connection, irrespective of location. It could revolutionize applications like remote healthcare, industrial automation, and emergency response systems.
This synergistic approach aims to create a more resilient and ubiquitous network infrastructure.
Implications for Industries
Industry | Potential Impact |
---|---|
Healthcare | Enhanced remote patient monitoring, real-time data transmission for surgeries, and telehealth services. |
Retail | Improved in-store customer experience through real-time data analysis and targeted promotions. |
Manufacturing | Real-time data collection for production processes, remote control of machinery, and automation. |
Education | Enhanced learning experiences through interactive content and virtual classrooms. |
Transportation | Improved connectivity for vehicles and traffic management systems, and support for autonomous driving. |
These are just a few examples of the transformative impact of future Wi-Fi standards on various industries. The evolution of Wi-Fi technology will undoubtedly continue to reshape the digital landscape, driving further innovation and development.
Concluding Remarks
In conclusion, History of Wi-Fi technology and its workings reveals a remarkable story of technological advancement. From its humble beginnings to its present-day applications, Wi-Fi has profoundly impacted our lives. The future looks promising, with emerging trends like Wi-Fi 6E and mesh networks poised to further enhance its capabilities and reach. This comprehensive overview provides a valuable understanding of Wi-Fi’s history and the key factors driving its ongoing evolution.
Post Comment