The Evolution Of Computer Processors What’S Coming In 2025
The Evolution of Computer Processors What’s Coming in 2025 sets the stage for a fascinating journey into the future of computing. This exploration delves into the historical evolution of processors, examining key advancements like transistors and integrated circuits, and their impact on speed and power. Current trends in processor design, including leading architectures and emerging technologies, are also explored.
The article then predicts future advancements, potential challenges, and the profound impact these innovations will have on emerging technologies like AI and machine learning.
The article will cover the anticipated improvements in performance, power efficiency, and thermal management in processors by 2025, along with potential breakthroughs in material science and manufacturing. It will also discuss potential new processor architectures and their applications. The impact on various sectors, including healthcare, finance, and entertainment, will be highlighted, and potential challenges, such as power consumption and thermal constraints, will be addressed.
Finally, the discussion will encompass future directions, from quantum computing to biological computing, and present speculative technologies and their potential impact on processors in the coming decade.
Historical Context of Processor Evolution
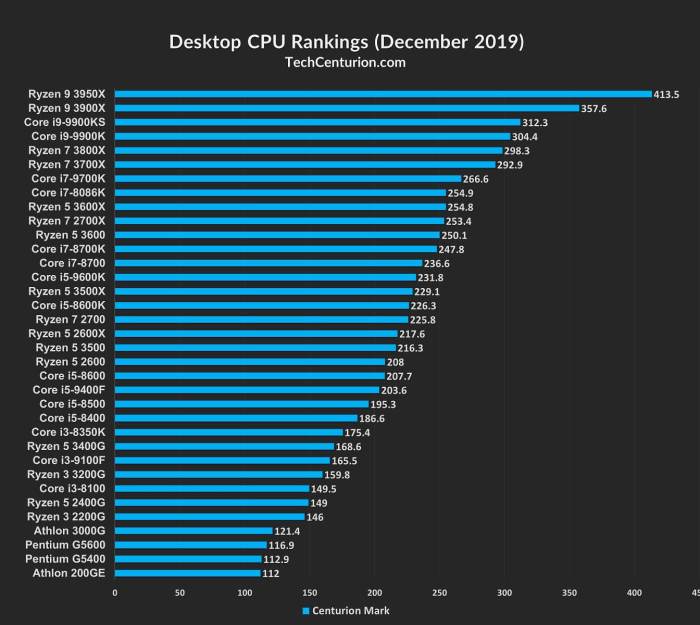
Source: techcenturion.com
The journey of computer processors has been one of remarkable progress, driven by relentless innovation and the pursuit of ever-increasing computing power. From bulky mainframes to the ubiquitous smartphones we carry today, the evolution of these components has profoundly shaped our world. This history is not merely a technical narrative but a reflection of societal progress and technological advancement.The initial designs were complex and required substantial resources.
The evolution of processor architecture was intricately linked with the development of supporting technologies, materials science, and the understanding of fundamental physical limits. This evolution has also led to a paradigm shift in how we interact with and use technology, impacting various aspects of modern life, from communication and entertainment to scientific discovery and industrial processes.
Key Advancements in Processor Architecture
The development of the transistor, a fundamental building block of modern electronics, marked a pivotal moment. Replacing bulky vacuum tubes, transistors enabled smaller, faster, and more reliable circuits. This advancement was crucial for miniaturizing computers and increasing processing speed. Integrated circuits, or microchips, further revolutionized the field by combining multiple transistors onto a single piece of silicon.
This integration dramatically reduced the size and cost of processors while increasing their computational capabilities. The advent of Moore’s Law, an observation that the number of transistors on a chip doubles approximately every two years, became a self-fulfilling prophecy, driving further innovation and development.
Generations of Processors and Defining Characteristics, The Evolution of Computer Processors What’s Coming in 2025
Different generations of processors have emerged, each characterized by specific advancements in architecture and technology. The evolution is a direct response to the ever-growing demands of computational tasks and the pursuit of faster, more efficient performance.
- Early Processors (1970s): These processors were characterized by limited transistor counts, resulting in relatively slow processing speeds and limited capabilities. The architecture was relatively simple, focusing on basic arithmetic and logic operations.
- Mid-Range Processors (1980s-1990s): The rise of personal computers brought about a significant increase in transistor counts and clock speeds. The architectures became more sophisticated, enabling more complex operations, including graphics and multimedia processing.
- Modern Processors (2000s-present): Moore’s Law continued to drive exponential growth in transistor counts, leading to dramatic increases in processing power. Multi-core architectures and advanced instruction sets (like SSE, AVX) became commonplace, allowing processors to handle multiple tasks concurrently. Energy efficiency and thermal management became increasingly important design considerations.
Comparison of Processor Generations
The table below illustrates the evolution of key processor features across different generations.
Generation | Transistor Count | Clock Speed | Architecture | Year |
---|---|---|---|---|
Early | Few thousands | MHz | Simple | 1970s |
Mid-Range | Hundreds of thousands | MHz to low GHz | Complex instructions | 1980s-1990s |
Modern | Billions | GHz | Multi-core, vector instructions | 2000s-present |
Current Trends in Processor Design
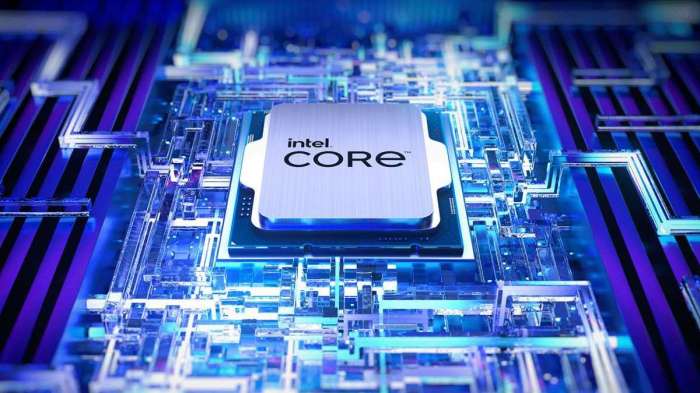
Source: tosshub.com
Modern processor design is a dynamic field, constantly evolving to meet the growing demands of computing applications. This evolution is driven by the need for higher performance, reduced power consumption, and improved thermal management. The ongoing race to develop more efficient and powerful processors fuels innovation across various architectural approaches and technological advancements.Current processor architectures are pushing boundaries in areas like heterogeneous computing and neuromorphic computing, reflecting a broader shift towards specialized hardware tailored for specific tasks.
This trend allows for more efficient resource utilization and improved performance in particular application domains.
Leading Processor Architectures
The two dominant processor architectures today are x86 and ARM. x86, largely synonymous with Intel and AMD processors, remains the prevalent architecture in personal computers and servers. Its extensive ecosystem of software and hardware support, coupled with its long history, provides a robust foundation. ARM, on the other hand, has become increasingly popular in mobile devices and embedded systems.
Its low power consumption makes it highly suitable for resource-constrained environments. The strengths and weaknesses of each architecture often dictate their best use cases.
Design Considerations for Modern Processors
Key design considerations in modern processors include power efficiency and thermal design. Power efficiency is crucial for portable devices and battery-powered systems. Minimizing power consumption while maintaining performance is a critical challenge. Thermal design is equally important, as excessive heat can damage the processor. Sophisticated cooling solutions are frequently integrated into modern systems to mitigate this issue.
Modern processor design often involves intricate thermal modeling and design strategies to optimize heat dissipation.
Comparison of Architectures
| Architecture | Strengths | Weaknesses | Use Cases ||—|—|—|—|| x86 | Extensive software ecosystem, high performance in general-purpose tasks, wide range of peripherals | Can be power-hungry, often more expensive | Desktop computers, servers, high-performance computing || ARM | Low power consumption, cost-effective, high performance in specific domains, suitable for mobile devices | Fewer software options compared to x86, might not be ideal for all general-purpose tasks | Mobile devices, embedded systems, IoT devices, some servers || RISC-V | Open-source, highly customizable, potentially optimized for specific use cases, flexible architecture | Smaller ecosystem compared to x86 or ARM, still developing | Specialized applications, embedded systems, high-performance computing with tailored needs |
Emerging Technologies
Heterogeneous computing involves combining different types of processors on a single chip, like CPUs, GPUs, and specialized accelerators. This approach allows for optimal resource allocation, leveraging the strengths of each component for specific tasks. Neuromorphic computing aims to mimic the structure and function of the human brain. This emerging technology has the potential to revolutionize machine learning and artificial intelligence applications.
Such specialized approaches can lead to significant improvements in performance and efficiency in certain areas.
Anticipated Advancements in 2025
Processor design is a dynamic field, constantly pushing boundaries in performance, power efficiency, and thermal management. Predicting precise advancements in 2025 is challenging, but based on current trends and ongoing research, we can anticipate several significant developments. The relentless pursuit of faster, more efficient, and cooler processors is driving innovation across various disciplines, including material science and manufacturing techniques.
Projected Improvements in Performance, Power Efficiency, and Thermal Management
Advancements in semiconductor fabrication techniques, such as extreme ultraviolet (EUV) lithography, are expected to allow for the creation of more complex and intricate transistor structures, leading to higher transistor density and improved performance. This translates to faster processing speeds and enhanced overall system performance. Simultaneously, optimized circuit designs and advanced caching strategies are expected to further boost performance.
Further improvements in power efficiency are also anticipated. Designers are actively pursuing techniques such as dynamic voltage and frequency scaling, along with low-power logic circuits, to minimize energy consumption without compromising speed. Simultaneously, advanced thermal management solutions, like innovative cooling systems and advanced thermal interface materials, will be crucial in mitigating heat dissipation challenges, crucial for maintaining reliability and performance under heavy loads.
Potential Breakthroughs in Material Science and Manufacturing
Material science plays a pivotal role in processor design. New materials with enhanced electrical properties, lower thermal conductivity, and improved durability are anticipated to emerge. For example, advancements in graphene or other 2D materials may allow for smaller, faster, and more efficient transistors. Innovative manufacturing processes, like those utilizing advanced nanofabrication techniques, will be essential in enabling the integration of these new materials and creating more complex processor architectures.
Improved techniques for deposition, etching, and doping will be key to realizing these advances.
Potential New Processor Architectures and Their Applications
New processor architectures, potentially based on neuromorphic computing or quantum computing principles, could emerge. Neuromorphic architectures, inspired by the human brain, are likely to excel in specific tasks like image recognition and natural language processing. Quantum computing, though still in its early stages, holds the potential to revolutionize certain computational tasks, but its application in consumer-grade processors is less imminent.
Hybrid approaches combining conventional and specialized architectures may also become prevalent, enabling processors to handle diverse workloads more efficiently.
Potential Processor Features for 2025
Feature | Description | Impact | Example |
---|---|---|---|
Enhanced Transistor Density | Increased number of transistors packed onto a chip | Higher processing speed and performance | 100 billion transistors on a single chip |
Advanced Cooling Mechanisms | Sophisticated thermal management systems | Improved reliability and sustained performance under heavy loads | Vapor chamber cooling with embedded phase change materials |
Neuromorphic Processing Units | Specialized units mimicking neural networks | Enhanced capabilities in AI tasks like image recognition and natural language processing | Real-time object detection in autonomous vehicles |
Hybrid Architectures | Integration of different processing units for diverse workloads | Increased efficiency in handling varied tasks | Combining CPUs with specialized accelerators for graphics or AI |
Quantum-Inspired Computing Elements | Incorporating quantum computing principles in certain areas | Potential to unlock new computational possibilities | Solving complex simulations or optimizations |
Impact on Emerging Technologies
Advancements in processor technology are poised to revolutionize various sectors, particularly those heavily reliant on computation. The increasing processing power and efficiency of these components directly impact the development and application of emerging technologies like artificial intelligence, machine learning, and high-performance computing. This influence is multifaceted, impacting not only the performance of existing applications but also fostering the creation of entirely new tools and capabilities.
Impact on Artificial Intelligence (AI)
AI algorithms, especially deep learning models, demand substantial computational resources. Modern processors, with their enhanced architectures and parallel processing capabilities, are crucial for training and deploying these complex models. Faster processing speeds allow for the development of more sophisticated AI systems capable of handling larger datasets and performing more complex tasks. This translates into improved accuracy and efficiency in applications ranging from image recognition to natural language processing.
Impact on Machine Learning (ML)
Machine learning models require significant computational power to analyze data and identify patterns. The increasing processing power of processors allows for the training of larger and more intricate machine learning models. This leads to more accurate predictions, better decision-making, and the development of new algorithms that can tackle complex problems in diverse fields.
Impact on High-Performance Computing (HPC)
High-performance computing relies heavily on specialized processors capable of handling massive workloads. As processors become more powerful and efficient, they enable the simulation of complex systems, the analysis of massive datasets, and the execution of computationally intensive tasks in areas like scientific research, financial modeling, and climate change prediction.
Impact on Healthcare
“Enhanced processor capabilities will lead to faster diagnostics and treatment options.”
Advanced processors are instrumental in developing sophisticated medical imaging systems and AI-powered diagnostic tools. They can process medical data in real-time, enabling faster and more accurate diagnoses. Examples include faster analysis of medical images, personalized treatment plans based on individual patient data, and the development of new drug discovery techniques.
Impact on Finance
“Increased computational speed in finance will improve risk assessment and portfolio management.”
The financial sector benefits significantly from faster processors that allow for the processing of vast amounts of financial data in real-time. This enables more accurate risk assessment, faster trading algorithms, and the development of sophisticated financial models. Predictive analytics, fraud detection, and algorithmic trading are examples of how processors influence financial decision-making.
Impact on Entertainment
“Higher processing power translates into richer and more immersive gaming experiences.”
The entertainment industry is experiencing a transformation as more powerful processors fuel the development of realistic graphics, interactive experiences, and virtual reality applications. The ability to process vast amounts of data enables more complex simulations and detailed visual representations in games, movies, and other forms of entertainment.
Challenges and Limitations: The Evolution Of Computer Processors What’s Coming In 2025
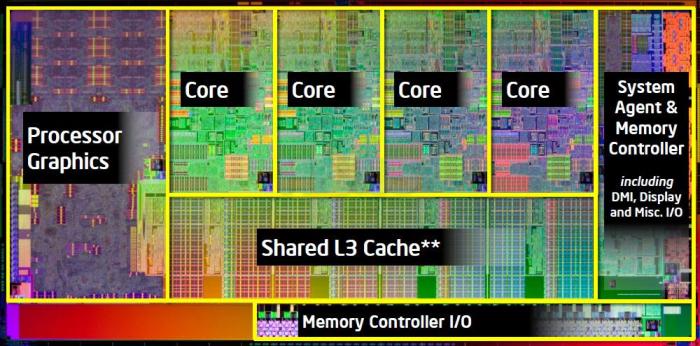
Source: hexus.net
Processor design faces a multitude of hurdles, constantly pushing the boundaries of what’s technically feasible. These constraints, from power consumption to material limitations, directly impact performance and applicability across various domains. Addressing these limitations is crucial for future advancements in computing.
Power Consumption and Thermal Constraints
Power consumption and thermal management are paramount concerns in processor design. Excessively high power demands lead to increased energy costs and generate substantial heat, potentially damaging the device and limiting its lifespan. Advanced cooling systems are required to maintain optimal operating temperatures, adding to the complexity and cost of production. Furthermore, the need to reduce power consumption is vital for portable devices and energy-conscious applications.
- High Power Density: Modern processors pack a significant amount of transistors into a small area, resulting in high power density. This high concentration of active components generates considerable heat, demanding sophisticated cooling mechanisms. For instance, the high power density in mobile processors necessitates innovative thermal solutions to prevent overheating and ensure long-term device reliability.
- Energy Efficiency: Improving energy efficiency is critical for battery-powered devices. Reducing power consumption while maintaining performance is a constant pursuit, driving research into novel transistor architectures and low-power circuit designs. The development of new materials and architectures, like those based on graphene or other 2D materials, promises to improve energy efficiency in the future.
- Thermal Throttling: Overheating can cause processors to throttle down their performance to prevent damage. This temporary reduction in performance can significantly impact applications requiring consistent high speeds, like video editing or gaming. Advanced thermal monitoring and control mechanisms are crucial to mitigate thermal throttling.
Material Limitations
The materials used in processor fabrication are crucial for performance, reliability, and scalability. Current silicon-based technology faces limitations in terms of scaling down transistor sizes and increasing speed. Discovering and implementing new materials with superior properties is essential to push the boundaries of processor capabilities.
- Material Properties: Silicon’s limitations in terms of electron mobility and heat dissipation are well-known. Alternative materials, such as gallium nitride (GaN) and graphene, offer potential advantages in terms of higher electron mobility and superior thermal conductivity, leading to improved performance and reduced power consumption. These materials could potentially replace or complement silicon in future processor designs.
- Manufacturing Challenges: Integrating new materials into existing fabrication processes presents significant challenges. Creating compatible fabrication techniques and addressing potential material defects is crucial to ensure consistent quality and reliability. New manufacturing techniques and approaches, like advanced lithography methods, are being developed to address these challenges.
Design Complexity
The increasing complexity of processor designs introduces challenges in terms of design verification, testing, and debugging. With billions of transistors, even a small defect can lead to significant performance issues. Developing sophisticated tools and methodologies to ensure design integrity and functional correctness is paramount.
- Verification and Testing: The sheer scale of modern processors makes traditional verification and testing methods insufficient. New techniques, such as formal verification and advanced simulation methods, are being employed to identify potential design flaws early in the development cycle. The use of AI and machine learning is emerging as a potential tool to accelerate the verification process and improve its accuracy.
- Design Space Exploration: Exploring the design space for optimal performance and power efficiency is becoming more computationally intensive. Employing machine learning algorithms to automate this process is expected to accelerate the development cycle and lead to more efficient designs.
Performance Comparison Table
Processor | Performance (Hypothetical) | Limitations | Solutions |
---|---|---|---|
Current Generation Intel Core i9 | High single-core performance, good multi-core performance | Power consumption, thermal throttling, limited transistor scaling | Advanced cooling solutions, power-efficient architectures, exploring new materials |
Hypothetical Processor 2025 (Based on Graphene) | Potentially higher performance, significantly lower power consumption | Material reliability, manufacturing complexity, integration with existing systems | Developing robust fabrication techniques, designing compatible interfaces, exploring advanced integration approaches |
Future Directions and Speculation
The relentless pursuit of faster, more efficient processors continues to push the boundaries of computing. While current trends focus on refining existing architectures, the long-term future holds the tantalizing prospect of entirely new paradigms, potentially revolutionizing how we interact with technology. This section explores potential future directions and intriguing possibilities in processor design.
Quantum Computing Approaches
Quantum computing promises to tackle problems currently intractable for classical computers. Quantum processors leverage the principles of quantum mechanics, exploiting phenomena like superposition and entanglement to perform computations in fundamentally different ways. This approach could lead to breakthroughs in fields like drug discovery, materials science, and artificial intelligence, where the complexity of the problems often exceeds the capabilities of classical computers.
Early quantum processors are still in their nascent stages, but the potential for transformative breakthroughs remains immense.
Biological Computing
Inspired by the remarkable efficiency of biological systems, biological computing explores the use of biological components like enzymes and DNA to perform computations. This approach could potentially lead to highly energy-efficient processors. While the practical implementation of biological computing is still a long way off, the fundamental principles offer an intriguing avenue for future processor design. The unique properties of biological molecules could allow for unparalleled levels of parallelism and adaptability.
Neuromorphic Computing
Neuromorphic computing aims to mimic the structure and function of the human brain. This approach focuses on creating processors that learn and adapt in ways similar to the human brain. This could lead to highly specialized processors for tasks like pattern recognition and decision-making, with the potential to significantly improve artificial intelligence applications. The design of neuromorphic chips often involves creating circuits that resemble the interconnected networks of neurons in the brain.
Speculative Technologies and Their Potential Impact
- Advanced Materials: The development of new materials with exceptional electrical conductivity and thermal properties could lead to processors with significantly improved performance and energy efficiency. Examples include graphene and other 2D materials, which could enable the creation of smaller, faster, and more powerful transistors.
- Adaptive Architectures: Processors that can dynamically adjust their architecture based on the task at hand could lead to unprecedented levels of performance. This approach could be particularly useful for handling complex tasks that require varying computational resources. An example would be a processor that automatically allocates more processing power to a computationally intensive task.
- Molecular Computing: The manipulation of individual molecules for computation is a futuristic concept. It could revolutionize computation by enabling incredibly small and energy-efficient processors, potentially leading to devices that are more efficient than current silicon-based processors.
- Hybrid Computing: Combining the strengths of different computing paradigms, such as quantum and classical computing, could yield a new generation of processors capable of handling a wider range of computational tasks. The potential impact would be substantial, allowing for efficient processing of both simple and complex problems.
Long-Term Implications
The long-term implications of these future directions are vast and multifaceted. From scientific breakthroughs to technological advancements, the evolution of processor design will likely reshape various aspects of our lives. The development of new computing paradigms could lead to a new era of scientific discoveries, unlocking solutions to complex problems in areas like medicine, materials science, and climate change.
The potential for more efficient and intelligent systems could lead to advancements in artificial intelligence and robotics, with significant implications for industries like manufacturing, transportation, and healthcare.
Illustrative Examples of Processor Advancements
Processor advancements are not simply incremental improvements; they represent revolutionary leaps in design and performance, often pushing the boundaries of what’s possible. These advancements, fueled by innovative architectural approaches and material science breakthroughs, have a profound impact on the technologies we use daily. This section provides concrete examples of processors that showcase these advancements, highlighting their benefits and drawbacks.
Early Examples: From the Transistor to the Microprocessor
The evolution from bulky, inefficient vacuum tubes to the miniature transistors and integrated circuits of modern processors is a remarkable journey. Early processors like the Intel 4004, a groundbreaking microprocessor released in 1971, demonstrated the potential of miniaturization and integrated circuits. The 4004’s design, while rudimentary by today’s standards, introduced the fundamental concept of a central processing unit (CPU) on a single chip, laying the foundation for all subsequent processor architectures.
Its limitations, however, were significant. Clock speeds were extremely slow, and memory capacity was limited, restricting its applications to basic calculations and control functions.
The Rise of Parallelism: Multi-Core Processors
The shift towards multi-core processors dramatically improved performance. Examples like the Intel Core i series demonstrate this transition. These processors leverage multiple processing cores, enabling simultaneous execution of tasks. This parallel processing approach significantly boosted performance in applications requiring substantial computational power, such as video editing and scientific simulations. However, managing the complex interactions between multiple cores and ensuring efficient data flow presented its own set of challenges.
Software optimization and design needed to adapt to harness the full potential of multi-core architecture.
Instruction Set Architectures: RISC and CISC
Different processor architectures, such as Reduced Instruction Set Computing (RISC) and Complex Instruction Set Computing (CISC), offer distinct advantages. ARM processors, based on the RISC architecture, are known for their efficiency and low power consumption, making them ideal for mobile devices. Intel processors, on the other hand, often employ a CISC design, providing a wider range of instructions for greater flexibility.
These choices influence both performance and power usage, leading to varying trade-offs in different applications. The optimal choice depends on the specific needs of the target application, balancing performance against efficiency.
Hypothetical Future Processor Design: The Neuromorphic Processor
Imagine a processor designed with a structure mirroring the human brain. A hypothetical “neuromorphic” processor would utilize interconnected “neurons” and “synapses” to perform computations. This approach, inspired by the human brain’s neural networks, could revolutionize machine learning and artificial intelligence applications. This hypothetical design could achieve extremely high levels of parallel processing, making it ideal for complex tasks.
The biggest hurdle, however, would likely be the immense complexity in design and manufacturing such a device. Current limitations in materials and fabrication techniques might restrict the development and widespread adoption of this type of processor in the near future. The benefits would be exceptional, enabling the creation of systems capable of mimicking human cognitive abilities.
Conclusive Thoughts
In conclusion, the evolution of computer processors is a dynamic field, promising remarkable advancements by 2025. From historical context to future predictions, this exploration highlights the incredible progress and the inherent challenges in this domain. The influence on emerging technologies is substantial, and the potential impact on society is profound. The future of computing is undoubtedly exciting, filled with possibilities and demanding careful consideration of both advancements and potential limitations.
Post Comment